Medical devices have become indispensable to everyday life and represent a growing market. Medical device applications range from huge machines (e.g. heart-lung machines) to diagnostic tools such as software and implants, hypodermic needles and mechanical contraceptives, to mention just a few. The compatibility of the materials utilized to create medical devices with the biological surfaces they encounter is of high importance, especially for the use of such devices on and inside the human body. Here, innovative materials offer new, advantageous properties, such as higher strength and better compatibility. Nanotechnology contributes to new product generations, e.g. by modifying the product surface with nanomaterials to improve compatibility or physicochemical properties.
What are medical devices and how are they defined?
Medical devices are strictly different from drugs or pharmaceutical products, as medical devices by definition do have no pharmacological or immunological effect and are not allowed to change their activity inside the body by adapted alterations (i.e. metabolism).
Medical devices are clearly controlled by EU regulation and the corresponding national implementation for each country
Medical devices can be very small or very big (from simple nanoparticles to joint implants or heart-lung machines). Regardless of their size, their compatibility must be guaranteed throughout their entire lifecycle.
Examples such as joint implants or heart valves illustrate clearly that such devices remain for long periods within the body and are in direct contact with the surrounding tissue. Thus, compatibility and safety of the materials which the medical devices are made from plays an important role.
For safety evaluations the medical devices are devided into two different categories:
- Non-invasive applications, e.g. products that contact the skin from the outside such as stethoscopes or mechanical contraceptives
- Invasive applications (through surgery or not) such as: materials for wound healing, implantable devices, dental- or bone cement, injectable materials
Innovative materials in medical devices
Innovative materials have become indispensable in the field of medical products. For example, the tiny batteries (e.g. for pacemakers) or circuits and sensors can often only be implemented with nanomaterials due to their reduced size. New ceramics for dental fillings or screws for dental implants, for example, consist of sintered or melted and re-solidificated (freezed) micro- or nanopowders (similar to 3D printing), other innovative materials such as specially adapted polymers or they have specially treated surfaces, so-called nanostructures.
Innovative materials
Today, a variety of innovative materials can be used in medical technology. Only a selection can be shown here. Many new and promising materials are being developed especially for medical applications, because on the one hand there are still no optimised treatment methods for many diseases and on the other hand the prospect of good sales is appealing.
A whole range of innovative medical device materials are shown at https://promatleben.de/de/ (inn German). The projects funded by the German Federal Ministry of Education and Research (BMBF) since 2018 in the “ProMatLeben – Polymere” initiative are explained here. The research field includes, for example, new polymers filled with active substances, polymer implant materials and coatings for implants. Innovative materials that encapsulate active substances and thus make them transportable in the body are called “carriers” in technical jargon; they are also explained on the website.
A frequently used innovative material for implants is titanium. Either as a pure metal or in the form of alloys with other elements such as aluminium, vanadium, nickel, niobium, molybdenum, etc., it is also used for implants. [1]. Such implants are always helpful in the human (and possibly also in the animal) body whenever structures need to be restored and stabilised.
Applications of these titanium materials range from ear implants to improve sound transduction [2], reconstruction of the eye socket for orbital fractures [3], dental implants [1], spinal implants [4], pelvic stabilisations [5] and stabilisations of broken limbs to foot corrections [6]. Here, titanium and titanium alloys are highly valued because of their high strength. In addition, the surface of titanium implants is coated with a micrometre-thick titanium dioxide layer, which is not recognised by the human body as extraneous and is therefore not repelled [6].
To avoid unnecessary follow-up operations, biodegradable materials are often used. In case of smaller implants, certain plastics but also magnesium are suitable for this. They can be degraded by the body’s own enzymes and then resorbed. [7].
Other materials such as cobalt-chromium alloys provide implants with even higher strength. However, such alternatives to titanium alloys can also have disadvantages, such as a higher allergy potential of the cobalt-chromium alloys [8]. Plastics, on the other hand, have significantly lower strength. That is why titanium implants have gained a high market penetration.
More recently, titanium materials have been joined by other moulded parts that must be described as innovative due to their novel production: 3D-printed titanium alloys (Ti- 6Al-4V)[ 4] produced with the help of electron beam melting can be manufactured as complex structures that are then used, for example, as cages in intervertebral disc operations to replace the disc.
Perovskites are currently being discussed as implant materials: Calcium titanate (CaTiO3) [9] [10] and barium titanate are interesting materials that can also be used in combination with fluorapatite (which largely corresponds to natural bone) [10] [11] or also plastics [12].
Nanomaterials
In addition to the examples of “large” medical devices, there are also very small ones. For example, iron oxide nanoparticles are an approved medical device for the physical treatment of tumours (e.g. in the brain), which are injected into the tumour and stimulated by a magnetic field to kill the tumour by overheating (hyperthermia) [13].
However, the iron oxide particles mentioned are only one example of the diverse applications of nanomaterials in this field. Even though much is still in the testing phase, there are various nanoscale materials that are already being used. Various ceramic but also plastic particles or carbon fibres in the nanometre range are used for bone reconstruction and dental prostheses. Nanosilver is used on the surfaces of implants or catheters to prevent or reduce inflammation, which can of course be very critical after an operation. However, nanoparticles or nanomaterials do not always have to be used so directly.
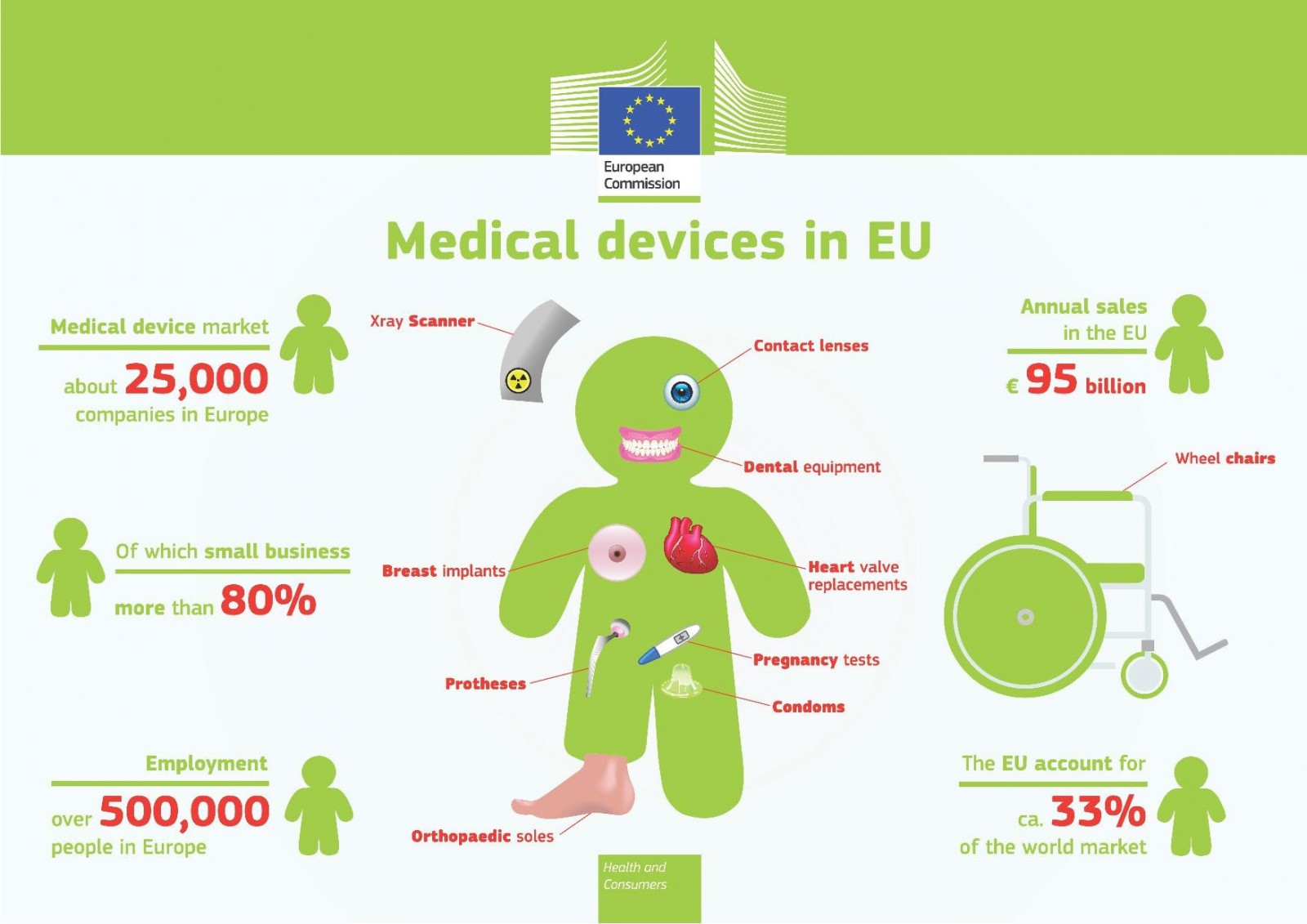
Medical products in Europe market volume (Quelle European Commission 2014)
Nano- and microstruktures
Nano- or microstructured surfaces can ensure improved incorporation of implants into the body [14] or better retention of the implant. Regardless of whether it is a screw in a bone, a dental implant in the mandible or a complete hip joint, inflammation must never occur and the inserted part should fulfil its task in the body for as “long as possible”. The surfaces of pacemakers should not and must not be recognised as being foreign bodies by the surrounding tissue, so that there is no unwanted rejection reaction by the body. For this reason, attempts are being made to improve the compatibility and significantly extend the service life of pacemakers with appropriate coatings and structures in the micro- or nanometre range.
Legal basis for the safety of medical devices
The legally binding bases for all medical devices are, as already mentioned above, the European Medical Device Regulation and the associated Medical Device Law Implementation Act. In addition to the definitions described above, medical devices are also divided into different categories according to how long they remain in or on the body. A distinction is made between short (less than one day), medium (between one and 30 days) and permanent residence time (longer than 30 days). The EU’s Committee on Newly Identified Health Risks has made these and other important classifications[15] and has set clear guidelines on how and what needs to be measured to ensure the safety of those medical devices that contain nanomaterials. In this context, testing for biological effects and toxicological reactions plays a very special role. A number of proposals have recently been made on how and what should be measured [16] [17] [18]. In principle, of course, medical devices should not release particles into the surrounding tissue, but nevertheless a test strategy must also be developed for such a case. In this context, it is sometimes also irrelevant whether or not micro- or nanoparticles are already contained in the initial product, because such very fine particles can also be produced by mechanical abrasion, e.g. in the case of a joint implant, and this should be tested beforehand or a possible health risk from these particles should be excluded as far as possible.
Medical devices are a broad and innovative field for new materials. Nanomaterials play an important role in improving the functionality of many medical devices, but can also be relevant for their tolerability. However, due to their use on and in the human body, they must meet very high safety requirements.
Literature:
- Revathi, A., et al., Degradation mechanisms and future challenges of titanium and its alloys for dental implant applications in oral environment. Mater Sci Eng C Mater Biol Appl, 2017. 76: p. 1354-1368.
- Schwab, B., R. Salcher, and M. Teschner, Comparison of two different titanium couplers for an active middle ear implant. Otology & Neurotology, 2014. 35(9): p. 1615-1620.
- Yi, W.S., et al., Reconstruction of complex orbital fracture with titanium implants. Int J Ophthalmol, 2012. 5(4): p. 488-92.
- Zippelius, T., et al., Safety and efficacy of an electron beam melting technique-manufactured titanium mesh cage for lumbar interbody fusion. Orthopade, 2019. 48(2): p. 150-156.
- Sturesson, B., et al., Six-month outcomes from a randomized controlled trial of minimally invasive SI joint fusion with triangular titanium implants vs conservative management. European Spine Journal, 2017. 26(3): p. 708-719.
- Matthews, M., et al., Long-Term Outcomes of Corrective Osteotomies Using Porous Titanium Wedges for Flexible Flatfoot Deformity Correction. J Foot Ankle Surg, 2018. 57(5): p. 924-930.
- Konneker, S., et al., Comparison of SCAphoid fracture osteosynthesis by MAGnesium-based headless Herbert screws with titanium Herbert screws: protocol for the randomized controlled SCAMAG clinical trial. BMC Musculoskelet Disord, 2019. 20(1): p. 357.
- Grupp, T.M., et al., Modular titanium alloy neck adapter failures in hip replacement–failure mode analysis and influence of implant material. BMC Musculoskelet Disord, 2010. 11: p. 3.
- Cheng, H., et al., Calcium titanate micro-sheets scaffold for improved cell viability and osteogenesis. Chemical Engineering Journal, 2020. 389: p. 124400.
- Coreño, J. and O. Coreño, Evaluation of calcium titanate as apatite growth promoter. Journal of Biomedical Materials Research Part A, 2005. 75A(2): p. 478-484.
- Jianqing, F., Y. Huipin, and Z. Xingdong, Promotion of osteogenesis by a piezoelectric biological ceramic. Biomaterials, 1997. 18(23): p. 1531-1534.
- Bagchi, A., et al., Perovskite ceramic nanoparticles in polymer composites for augmenting bone tissue regeneration. Nanotechnology, 2014. 25(48): p. 485101.
- Maier-Hauff, K., et al., Efficacy and safety of intratumoral thermotherapy using magnetic iron-oxide nanoparticles combined with external beam radiotherapy on patients with recurrent glioblastoma multiforme. Journal of Neuro-Oncology, 2011. 103(2): p. 317-324.
- Bruinink, A., et al., Addition of nanoscaledbioinspiredsurface features: A revolution for bone related implants and scaffolds? Journal of Biomedical Materials Research Part A, 2014. 102(1): p. 275-294.
- Commission, E., D.-G.f. Health, and Consumers, Guidance on the determination of potential health effects of nanomaterials used in medical devices. 2015: European Commission.
- Gubala, V., et al., Engineered nanomaterials and human health: Part 1. Preparation, functionalization and characterization (IUPAC Technical Report). Pure and Applied Chemistry, 2018. 90(8): p. 1283-1324.
- Gubala, V., et al., Engineered nanomaterials and human health: Part 2. Applications and nanotoxicology (IUPAC Technical Report). Pure and Applied Chemistry, 2018. 90(8): p. 1325-1356.
- Myers, D.K., et al., From in vivo to in vitro: The medical device testing paradigm shift. ALTEX – Alternatives to animal experimentation, 2017. 34(4): p. 479-500.