Plastics are materials that consist of single styrene molecules bonded together to form long chains, so-called “polymers”. They are produced in chemical reactors. Because of their properties, these plastics can be extruded, moulded, or pressed into various objects. One of the most commonly used plastics is polystyrene, which is economical and lightweight. Polystyrene is made by the controlled linking of single styrene molecules. Nanoscale polystyrene particles can be synthesized in the laboratory. They are called primary polystyrene nanoparticles. Nanoscale polystyrene particles produced from polystyrene waste through degradation and abrasion, on the other hand, are called secondary polystyrene nanoparticles.
How can I come into contact with this material?
Since polystyrene is used in large quantities in everyday objects such as such as yoghurt containers, plastic tableware or as packaging, it is found as waste in the environment. However, an assessment of how much polystyrene particles come into contact with humans has not yet been carried out. Research is currently being conducted on this topic (2021).
Is there any risk from this material to humans and the environment?
Pure polystyrene nanoparticles are considered to be non-toxic and of low concern to the organism. In research, they are mostly used as non-toxic reference or model particles for in vitro or in vivo studies.
Conclusion
Humans and the environment are in constant contact with polystyrene. In its meso or micro form, it is of particular concern for aquatic life, but polystyrene does not play a special role in human health. Research on this topic is currently being carried out.
By the way
Polystyrene products can be recognized by the imprint 6 in a triangular arrow frame and the abbreviation PS underneath.
Properties and Applications
Plastics are a wide range of artificial materials whose main component comprises polymers. Their plastic property makes it possible to mould, extrude or press them into solid objects of different shapes. One of the most common plastics is Polystyrene due to its low cost and weight. This naturally transparent polymer is composed of many styrene units, the so-called monomers.
At room temperature, bulk polystyrene is generally amorphous, stiff, and non-reactive. It is insoluble in water and very stable against bases or acids. Varying polymer chain properties such as the length of the chain (number of styrene units) can lead to different materials which make it possible to form products that range from cutlery to petri dishes used in laboratories worldwide. Polystyrene can also be found as foam comprising up to 95-98% air. These polystyrene foams are excellent insulation materials that tend to have a very long duration of use.
Polystyrene particles below 1 micrometre in size, are used in a wide variety of applications within scientific research. The properties of these nanoscale polystyrene particles can be highly tailored. Therefore, polystyrene nanoparticles are being investigated for applications ranging from components for electronic devices to dyes. Moreover, due to their ability to encapsulate other substances and their high adsorption affinity of various molecules and proteins on their surface, new potential applications in the medical field as nanoscale carriers for drugs are being studied.
Whereas polystyrene particles at the nano or micro-scale synthesized in the laboratory are considered a new, advanced material, these are also unintended by-products of irresponsible handling of plastic waste. Polystyrene products discarded in the environment can be degraded by ultraviolet light (e.g., contained in sunlight) and mechanical forces such as waves crashing onto a beach. Once broken down, the products become a source of polystyrene microparticles and, potentially, nanoparticles. Because polystyrene litter is nearly everywhere in the sea, it can be taken up by various animals and thus enter the food chain and end on our plates.
Origin and production of polystyrene
Large scale production of polystyrene products makes it almost impossible to make it through your day without encountering this plastic in some form. The different sorts and sizes of polystyrene are not made through natural processes, all polystyrene is the result of human-made sources. Briefly, polystyrene results when styrene monomers are linked together by the controlled addition of monomers in a specific amount and order in a process known as polymerisation.
Nano- and microscale polystyrene particles are available from a variety of companies and can be purchased commercially for use in laboratory experiments. Nonetheless, these particles are easy to produce and there are a variety of possible synthesis protocols. Therefore, they are usually produced in-house in most research institutions.
Once a polystyrene product has reached the end of its use, it is often burned or discarded and taken to landfills or recycling plants for disposal. Unfortunately, it is also common for these products to be discarded directly into the environment as litter. Polystyrene can float on water and therefore can be carried downstream in rivers or pulled out to sea on ocean waves. These plastic products are weathered and broken into smaller pieces by UV light from the sun and by mechanical forces from wind, water currents, or waves.
The first step of this breakdown process usually results in polystyrene fragments measuring between 20 centimetres and 5 millimetres, also called mesoplastics. Mesoplastics further break down into small particles called microplastics (5 millimetres to 1 micrometre). Microplastics can potentially break down further into nanoplastics (below 1 micrometre), but there are several limitations in both sampling and detection techniques that make studying these particles environmentally very challenging.
The gradual breakdown of polystyrene particles from millimetre to micrometre and nanometre sizes leads to unintentional ingestion by fish and other marine life. So, when people eat fish or seafood from open waters, polystyrene is most likely part of that dish.
In contrast, polystyrene nanoparticles produced in the laboratory do not enter the food chain because laboratory waste is incinerated or sent to landfills.
Further information
Polystyrene nanoparticles are considered to be non-toxic and of only low concern to the body. They can enter the environment by degradation processes of polystyrene (plastic) products. In research they are mostly used as non-toxic reference or model particles in in vitro or in vivo studies.
Everyday contact
Polystyrene (not nano) is found in many everyday applications. The material is popular for many applications because it is usually neither toxic nor biodegradable - so it is biocompatible and stable.
The most likely way to ingest polystyrene nanoparticles is usually from food. They may come from plastics in the environment and thus enter humans via the food chain. There are currently no data on nanoplastics in food, partly because detection and differentiation from other substances is as yet almost impossible . However, special methods for this are constantly being improved .
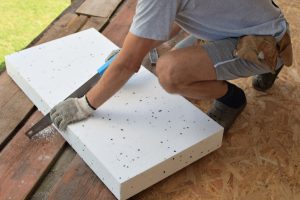
Craftsman cutting polystyrene panel © Inga - stock.adobe.com
Situation at the workplace
During polystyrene production and processing, polystyrene nanoparticles can be inhaled at the workplace. Due to their size, the nanoparticles can reach the deep lung regions after inhalation and may pass into the bloodstream. Polystyrene nanoparticles are not toxic, do not trigger inflammatory reactions and are therefore considered to be harmless.
Consumer situation
Polystyrene (non-nano) is found in many everyday products. It is one of the standard plastics for food packaging, for example as yoghurt pots or foam trays or transparent film for packaging purposes. It is physiologically harmless and approved without restriction for food packaging. In the form of foam, it serves as a lightweight packaging and insulation material.
Polystyrene nanoparticles as model particles in the biology laboratory
Although polystyrene nanoparticles can enter the body via inhalation or ingestion, they are considered to be non-toxic and not very harmful to the body. They are therefore used as model particles in numerous in vitro and in vivo studies. The particles can be loaded with special substances, for example, in order to be taken up efficiently by cells. In these studies, it is not important to model actual exposure of the body to polystyrene nanoparticles, so very high doses are quite often used. These particles have the advantage of being available on the market in different varieties, sizes or surface coatings. Loaded with fluorescent dyes, the particles can be visualised and tracked by fluorescence microscopy .
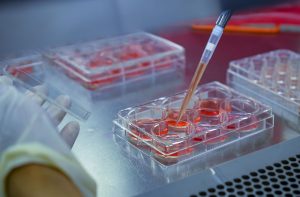
Filling a liquid into a petri dish with a pipette © manjurul haque/EyeEm - stock.adobe.com
Like almost all nanoparticles, polystyrene nanoparticles bind biological substances to their surface, especially proteins. This creates a shell around the nanoparticles that normally makes them less harmful. This is also confirmed by a broad toxicity study with coated polystyrene nanoparticles. In the absence of proteins, the analysed cells only showed toxic effects at 2.5-fold the amount (EC50 value) of polystyrene nanoparticles. Since proteins and other biomolecules are always present in the human body, this protective effect is automatically present .
Methods using intact organs are intermediate steps to animal experiments. This is particularly interesting for the heart, as its functions are difficult to imitate in cell culture experiments. Experiments with hearts from guinea pigs showed no impairment of heart function when exposed to polystyrene nanoparticles in contrast to e.g., other particles from combustion processes .
Numerous studies on the uptake, distribution and effect of polystyrene nanoparticles use injection in a living organism. The aim here is to investigate particle candidates for medical use. The field of nanomedicine is heavily researched and has already brought drugs, diagnostics or implants (see also Nanomaterials in Medical Devices) into the clinic. Particles are a means to an end here because they can deliver their cargo to the desired cells or tissues better than would be possible without their help. It is also often useful that particles can be easily loaded with several substances at the same time. However, due to their large surface area, nanoparticles can also have non-specific effects, as demonstrated by the impairment of iron absorption in the intestine of chickens .
Nanoparticles made of polystyrene are often used in nanomedical research. However, it is entirely possible that they will be replaced by other particles for the treatment of patients in further development. Chemically coated polystyrene nanoparticles have been shown to cause pseudoallergy in pigs. Since this type of reaction is a known problem for drugs, the particle variant in question is probably not a promising candidate. However, polystyrene nanoparticles do not trigger inflammation unless they have been specifically modified for this purpose .
Human contact with polystyrene particles is low. If there is, there could be exposure at the workplace by inhaling powder, or via ingestion of polystyrene contamination of food. Polystyrene nanoparticles are not harmful, but substances bound to their surface may be a problem. Polystyrene nanoparticles are mainly used as model particles to study transport processes in cells or the body.
Polystyrene nanoparticles enter the environment either as deliberately engineered polystyrene nanoparticles (as so-called primary polystyrene nanoplastics) or as polystyrene debris that forms nanoscale particles due to polymer degradation, abrasion and fragmentation of larger polystyrene items (so-called secondary polystyrene nanoplastics).
Release of polystyrene nanoparticles
Engineered polystyrene nanoparticles are intentionally used as standards for laboratory and biomedical applications. In addition, non-nano polystyrene composites are applied in food packaging (see cross cutting article - Nanomaterials in food packaging). These applications may potentially lead to an environmental release during usage as well as disposal of the products. Once in the environment, it is challenging to differentiate between primary polystyrene and secondary polystyrene particles .
Engineered polystyrene nanoparticles are often used in laboratory studies as a non-toxic representative for nanoparticles in general. They are used as calibration material for various analytical methods or in combination with specific dyes for microscopic applications. That way, the interaction of the polystyrene nanoparticles with environmental organisms, uptake behaviour, location within the organisms and even cells can be studied in detail.
The release of polystyrene nanoparticles is most likely to occur by direct release into water or littering, and into soils via sludge as well as littering. Polystyrene particles in various size ranges will continue to be present in the environment, since polystyrene is one of the most commonly detected type of environmental plastic debris. Unlike other polymers, the abundance of polystyrene particles (nano and non-nano) is not differing between water and sediment phase, due to the two distinct forms of polystyrene. The solid PS with a density higher than water will presumably sink into the sediment, whereas the PS foams with a density lower than water will rather float .
Polystyrene nanoparticles have been detected in soils . In the atmosphere, polystyrene microparticles have been detected, and based on this finding it is predicted that also polystyrene nanoparticles occur .
Released amount of polystyrene nanoparticles
Polystyrene nanoparticles in the environment are expected to occur predominantly in the form of secondary polystyrene nanoplastics. However, actual measured environmental concentrations are not yet available. Currently the sum of all nanoplastics can be detected, but it is impossible to differentiate between different types of polymers . Hence, estimated exposure concentrations are not available specifically for polystyrene nanoplastics .
This is mainly due to methodological challenges associated with the extraction and detection of small carbon-based particles in complex natural matrices (see cross cutting article - Detecting nanomaterials in the environment). Furthermore, it is not yet possible to differentiate between engineered polystyrene nanoparticles and secondary polystyrene nanoparticles in the environment .
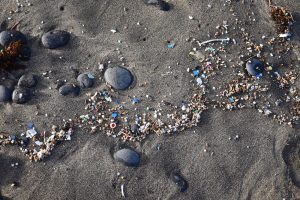
Plastic particles which have been found on the beach © Susanne Fritzsche - stock.adobe.com
Polystyrene currently accounts for 6.7 % of the total plastic demand in Europe. Based on a total European annual plastic production of 60 million tonnes in 2016, this corresponds roughly to an annual production of 4 million tonnes polystyrene. This equals the production of 2.2 million of yogurt cups per minute in Europe! However, it is unknown what share is produced as nanoparticles, and what share of polystyrene is released into the environment, and how long it takes to degrade the mismanaged plastic items into secondary polystyrene nanoplastics in the environment .
So far, polymers are excluded from registration under the European chemical regulation (REACH), this practice is currently under discussion and criteria important for a registration of polymers were suggested (ICPC, 2021). Even if polymers would require registration, for engineered polystyrene nanoparticles this would not be the case because the production volume is too low to exceed the tonnage requirements.
Laboratory results on the release of polystyrene nanoparticles
There are no estimations of primary polystyrene nanoparticle release available. As well, secondary polystyrene nanoparticle generation from larger polystyrene items has not yet determined under environmental conditions, but it is known to occur under laboratory conditions .
A very sensitive methodology for nano polystyrene detection has been developed. It requires the labelling of particles with a specific form of carbon, and hence does not allow direct measurement of nanoplastics in the environment because they do not carry the label. However, the method can be used to better understand the distribution of nanoplastics in the environment and in organisms in laboratory experiments (see spotlight article) .
In summary, large production volumes and usage of polystyrene particles in many products imply environmental release and hence exposure. However, environmental concentrations for polystyrene nanoparticles are hard to estimate for primary as well as for secondary nanoparticles. The fragmentation of larger polystyrene items into nanoparticles has so far only been demonstrated under laboratory conditions.
Further information
- European Chemicals Agency (2019). Appendix for nanoforms to the guidance on registration and the guidance on substance identification. ISBN978-92-9481-357-2, DOI: 10.2823/832485
- PlasticsEurope (2020). Plastics - the Facts 2020 - an Analysis of European Plastics Production, Demand and Waste Data. (PDF, 4 MB)
Polystyrene nanoparticles can be inhaled. They can also enter the body via food. Healthy skin, on the other hand, is a good barrier for these particles.
Uptake via the lung
The uptake of polystyrene nanoparticles via the lung may play a role primarily at the workplace. Nanosized plastic particles occurring in the environment can also be inhaled. However, the quantities of these secondary polystyrene nanoplastic particles are very low. Furthermore, there are approaches in medicine to use polystyrene nanoparticles as a means of transport for the administration of medicines via the lung.
In vitro experiments have shown that polystyrene nanoparticles are taken up by epithelial cells and macrophages of the lung, but do not cause any negative effects. In vivo, polystyrene nanoparticles administered by instillation also do not cause toxic effects or inflammatory reactions in rats. The immune system thus considers the polystyrene nanoparticles to be harmless.
In the alveoli, the inhaled polystyrene nanoparticles initially come into contact with the lung surfactant. This fluid is responsible for maintaining surface tension in the alveoli and is made up of 90% lipids and protein complexes with a large lipid content. These surfactant-protein complexes form a protein shell around the polystyrene nanoparticles, which usually has a mitigating effect on the potential effects of the nanoparticles. Studies on an in vitro infection model of lung cells have shown that the loading of the polystyrene nanoparticles with surfactant-protein complexes has a dose-dependent influence on the control of influenza viruses. Low concentrations of the loaded polystyrene nanoparticles inhibited the fight against influenza viruses, while higher concentrations promoted it. Whether this effect can also occur in the living organism is not yet known zotpressInText item="{4274171:BIXJKYFE},{4274171:G5L9CL49},{4274171:BV6L2TKT},{4274171:884S6UAI}"].
Uptake via the skin
Skincare and cosmetic products bring the skin into contact with larger polystyrene particles (not nano). Although polystyrene nanoparticles are not considered critical, they are not currently approved for use in cosmetic products in the EU.
If the skin is healthy, the polystyrene nanoparticles cannot overcome this protective barrier and enter the body (cf. body barriers - nanoparticles and the skin). It has been shown that polystyrene nanoparticles can penetrate hair follicles, but no effects could be demonstrated.
The question of how damaged or injured skin deals with nanoparticles is discussed again and again. An in vitro model of human corneal cells has shown that polystyrene nanoparticles can slow down wound healing in the case of injuries. However, it is not known whether this effect also occurs in reality for normal skin, i.e. on living organisms. .
Uptake via the gastro-intestinal tract
Technically produced polystyrene nanoparticles, as well as secondary polystyrene nanoparticles, can be taken up into the human body via food. They are considered uncritical, but there is a lack of data, especially on the long-term effects of secondary polystyrene nanoparticles.
Uptake via food is considered the most important uptake route for polystyrene particles in the human body. There are no data on nanoplastics in food, which is also due to the fact that detection and differentiation from other substances is hardly possible so far .
Studies on the uptake and effect of polystyrene nanoparticles have so far only been carried out with model particles. Such technically produced polystyrene nanoparticles have a uniform size, shape and purity.
A series of tests on chicken chicks showed that two weeks of administration of polystyrene nanoparticles impaired iron absorption in the intestine. Feeding polystyrene nanoparticles to rats over a five-week period resulted in no significant changes in animal behaviour in a series of tests .
There are currently no data on real long-term effects (over years) for animal models of human health. It is not known whether the particles remain in the intestinal lumen or will be taken up into the body via the intestinal barrier. There are animal studies that have shown an accumulation of polystyrene nanoparticles in Peyer's patches, a collection of lymphoid follicles in the intestine. However, effects have not been described.
Uptake via medical application
The fact that polystyrene nanoparticles can be taken up by cells leads to their medical application, e.g., as carriers for other substances whose effect is improved by binding to particles. One new possibility for cancer therapy, for example, are the so-called "checkpoint inhibitors", for whose scientific discovery the Nobel Prize in Medicine was awarded in 2018. These substances do not target the tumour, but the immune system, which is induced to attack the tumour. In animal experiments, it has already been shown that the effect is significantly better when the drugs are bound to polystyrene nanoparticles (e.g. ). The majority of the analysed applications, like this one, focus on cancer. So far, polystyrene nanoparticles have not been used clinically, but efforts are underway in this direction, including for other diseases. A particularly interesting possibility for stable particles such as polystyrene nanoparticles is oral administration, because such particles survive the extremely acidic environment in the stomach well.
Polystyrene nanoparticles do not cause any toxic effects in the human body. However, they can impair the absorption of other substances in the intestine, such as iron. Long-term effects need to be analysed in more detail.
Engineered polystyrene nanoparticles have been subjected to laboratory studies in a variety of test organisms to unravel their potential hazardous effects. They are ingested by different environmental organisms and can adhere to the body surface of organisms. Laboratory studies indicate a potential transfer of polystyrene nanoparticles from primary consumers through food chain to predators. The biological effects are found to depend on particle properties such as surface charge, coating and size.
Release of polystyrene nanoparticles in environmental organisms
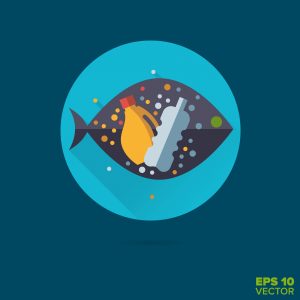
Illustration of a fish which swallowed plastic bottles and plastic particles © eyewave - stock.adobe.com
Many test organisms ingest polystyrene nanoparticles in laboratory studies (e.g. mussels, daphnids). Under environmental conditions such uptake has not yet been proven but is very likely to occur as well. Polystyrene nanoparticles adhere to external body surfaces of aqueous animals, (e.g. crustaceans) which may result in impaired swimming and feeding. As well, adherence to algae and water plants was observed . Fluorescent polystyrene nanoparticles are used in many studies to investigate the body distribution of the particles. Fluorescence signals were detected inside zebrafish embryos, water flea and nematodes, and transfer of polystyrene nanoparticles across tissue barriers, such as the blood-brain barrier and the gut, has been assumed . In addition, transfer from mother to the offspring has been reported in zebrafish . Recently, however, fluorescent dye was shown to leach from polystyrene nanoparticles resulting in the false impression that particles are transported . Hence, it needs to be proven by future studies, whether polystyrene nanoparticles are able to cross tissue barriers.
Toxicity of polystyrene nanoparticles in environmental organisms
Polystyrene nanoparticles exerted no or moderate effects on the viability of different animals (fish, water flea, nematodes, mussels, plants) when exposed for short periods of time (up to 3 days) . Upon longer exposure periods, effects such as increased mortality in crustaceans and marine rotifers, altered swimming behaviour of fish larvae or alteration in the filtering activity of mussels were observed . In crustaceans, sea urchins, mussels as well as nematodes, longer exposure to polystyrene nanoparticles resulted in reduced reproduction . In addition, polystyrene nanoparticles induced oxidative stress in adult fish, sea urchins, oysters, crustaceans, nematodes, and plants . Polystyrene nanoparticles affect plants by reducing shoot and root growth at high exposure concentrations . Most of these laboratory experiments used particle concentrations far above those expected in the environment, and many studies conclude that effects observed are not expected to be elicited at environmentally relevant concentrations.
The observed effects depend on the charge of nanoparticles. Most effects in mussels occur for positively charged particles . For oyster and algae, however, negatively charged particles had more severe effects . Upon addition of humic acid to the test mixture simulating environmental conditions, the toxicity of polystyrene nanoparticles towards water fleas was diminished .
Interestingly, in zebrafish and nematodes polystyrene microparticles showed stronger effects compared to polystyrene nanoparticles. In contrast, the opposite effect was observed in algae and water flea, with the nanoparticles exerting more severe effects compared to the polystyrene microparticles . No clear effects occurred in nematodes, where the small nanoparticles and the larger microparticles were similarly toxic, but particles in intermediate sizes were the most toxic . Taking together all results obtained in aquatic and marine organisms on nanoplastic in general into consideration, nanoplastic particles were found to be less toxic than microplastic, as well as other engineered nanomaterials .
It is important to mention that chemicals used as dispersant aids for polystyrene nanoparticles were shown to have adverse effects . This needs consideration for hazard assessment.
Assessment of the effects of polystyrene nanoparticles in environmental organisms
It is not yet possible to set toxicity data obtained in laboratory studies in relation to environmental concentrations of polystyrene nanoparticles. First, environmental nanoplastics concentrations are generally difficult to determine. Second, most environmental polystyrene particles will be secondary particles differing in their characteristics from the primary ones used in laboratory studies. It is therefore not possible at present to assess the risk posed by polystyrene nanoparticles and to distinguish it from the risk posed by the mixture of various micro- and nanoplastic particles present in the environment.
In summary, engineered polystyrene nanoparticles are internalised by various environmental organisms. They do not exert very severe acute effects but provoke sublethal effects after longer exposure periods. The polystyrene nanoparticles adhere to body surfaces of organisms and impair their normal functioning. Their effects on environmental organisms depend on surface charge with some studies providing clear evidence that positively charged nanoparticles provoke higher effect. In some organisms, the effects of nanoparticles differ from those of microscale polystyrene.
Polystyrene nanoparticles can be taken up into cells and overcome body barriers via a range of uptake mechanisms.
Distribution and effects in the body
Medical applications and experimental studies with particles usually work with high concentrations that are far above what can enter the body via unintentional uptake through the digestive tract, respiratory tract or skin . With regard to medical applications, it is important to know whether the particles preferentially bind to specific cells or tissues so that drugs can be specifically enriched at the desired site of action. This is often achieved by applying substances to the particles' surface that only find binding partners there. The shape may also play a role: oblong polystyrene nanoparticles, in contrast to spherical ones, accumulate in the lung and bind preferentially to inflamed sites.
Behaviour at the blood-brain barrier
Polystyrene nanoparticles accumulate better in the brains of mice when inflammation is present there . This property is very desirable when it comes to delivering drugs to inflamed blood vessels in the brain, for example in cases of stroke. There, the passage through the blood-brain barrier is then also facilitated.
Behaviour at the placental barrier
Nanoparticles may be able to cross the placental barrier and reach the embryo. It is therefore important to understand the mechanisms responsible for this. Knowledge of this would aid the development of appropriate drugs and allow prediction of which nanoparticles this might be a problem for. Studies on this are difficult because the placenta is a very complex organ and also very variable in different species. It cannot be completely reproduced in cell culture. Studies with polystyrene nanoparticles on human placenta obtained after caesarean sections provide initial indications: A specific placental layer - the trophoblast - was decisive for the uptake of the nanoparticles studied. Transport across this barrier occurred via as yet unidentified, active mechanisms, possibly also via phagocytosis, since relatively large particles can be transported. It is possible that uptake is lower during most of pregnancy because this barrier only becomes thinner towards the end of pregnancy .
Uptake and effects in cells
In principle, nanoparticles can be taken up via a range of normal cellular uptake mechanisms. Which pathways are utilised is dependent on the cells used, but also on the size, surface area and surface charge of the particles. Generally, uptake into cells occurs via active uptake.
It should be noted that the uptake of nanoparticles into the cells changes when the cells grow in a three-dimensional cell culture (co-culture) in which they are in contact with other cells in every direction. This corresponds to the conditions in the body. However, this does not apply to normal, two-dimensional cell culture, where cells grow only on the bottom of a culture vessel. For polystyrene nanoparticles, it has been shown that cells in three-dimensional culture take up these particles more slowly than in two-dimensional culture. The most important uptake pathway is pinocytosis. Polystyrene nanoparticles labelled with fluorescent dyes can also be observed inside the cell. After uptake into vesicles, they migrate via endosomes and lysosomes, thus passing through a series of cell organelles, as do many other ingested substances .
Polystyrene nanoparticles can be taken up into cells and overcome body barriers.
Polystyrene nanoparticles occur in the environment mainly as secondary polystyrene nanoplastic particles formed through the fragmentation of larger polystyrene items. How these particles distribute and transform in the environment depends on their properties as well the interaction with organic materials and biota.
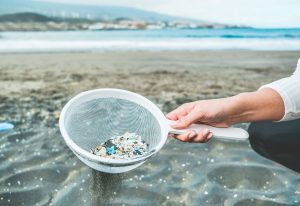
Granulated plastic particles, collected from the ocean © DisobeyArt - stock.adobe.com
Transport
In water, the density of polymeric materials will decide on the transport behaviour. The solid polystyrene with a density higher than water will presumably sink into deeper layers of water and sediment. The polystyrene foams with a density lower than water will rather remain on the water surface .
Like for other nanomaterials, the surface charge has been shown to play a role in the behaviour of polystyrene nanoparticles in water. Engineered polystyrene nanoparticles with a positive surface charge are stable in pure water, meaning there is no agglomeration and sedimentation occurring. In natural waters, however, the engineered polystyrene nanoparticles acquire a negative surface charge and subsequently form larger aggregates with natural organic matter and other particles. In seawater, larger aggregates are formed compared to fresh water due to the higher salt content. Hence, no single nanoscale polystyrene particle will occur in a real-life environmental scenario .
The majority of environmental polystyrene nanoparticles are expected to originate from fragments of larger polystyrene items, e.g. discarded coffee cups. During their residence time in the environment, these items fragment into smaller and smaller particles under the influence of sunlight, wind and waves (weathering), ultimately leading to the formation of nanoparticles. Little is known on the transport behaviour of secondary polystyrene nanoparticles. Some knowledge, however, can be derived from larger polystyrene microplastic particles. Upon entrance into environment, microplastic particles are quickly coated with organic material (e.g. humic acids) and microorganisms (eco-corona or biofilm formation). This organic coating changes the buoyancy of the particles as it increases its density causing otherwise floating particles to sink to the ground. Likewise, weathering of polystyrene microplastics by UV irradiation and mechanical forces increase the sinking rates as well as the adsorption of pollutants. It is assumed that the described processes will also apply to polystyrene nanoparticles .
Little is known about the transport of polystyrene nanoparticles in soils for example in agricultural areas .
Transformation
It is assumed that primary and secondary polystyrene nanoparticles undergo further fragmentation and degradation in the different environmental compartments. With current analytical methods, however, it is difficult to detect polystyrene nanoparticles in water, air and soil. Furthermore, it is not yet possible to differentiate between engineered polystyrene nanoparticles (primary) and secondary polystyrene nanoparticles in the environment (see cross cutting article - Detecting nanomaterials in the environment).
So far, the fragmentation process of polystyrene into secondary polystyrene nanoparticles has only been studied in laboratory experiments (see cross cutting article -Nanoplastic in the environment) . It is unknown in which time scales secondary polystyrene nanoparticles are formed under environmental conditions. It is assumed that complete degradation (mineralisation) of polystyrene nanoparticles is possible, and it has been demonstrated that microorganisms are capable of degrading polymers, however in the specific case of polystyrene nanoparticles, no data are available.
In agricultural areas, polystyrene nanoparticles will also enter soils. Most secondary polystyrene nanoparticles will presumably remain in soil for many decades. Compared to the aqueous environment, degradation in soil is slower and might as well be enhanced by microorganisms. However, other processes which may occur for polystyrene nanoparticles and their transformation in soil is currently unknown .
Most knowledge on the environmental behaviour of polystyrene nanoparticles is derived from larger, microplastic polystyrene particles. Up to now it is hardly possible to sample, detect and analyse polystyrene nanoparticles under environmental conditions. In an aqueous environment, the nanoparticles are quickly coated with organic material affecting the particles’ flotation behaviour. There is limited knowledge on the behaviour of polystyrene nanoparticles in soils.