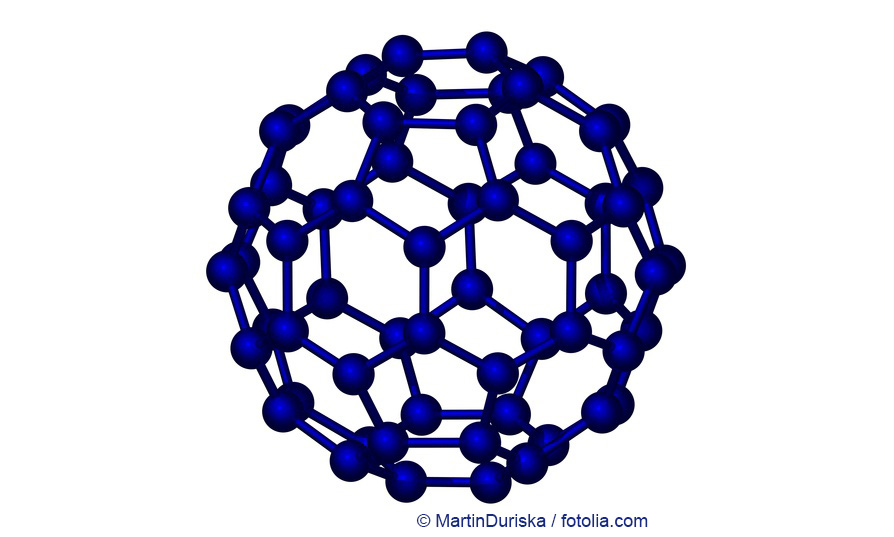
Buckminsterfullerene © Martin Duriska / fotolia.com
Fullerenes, also called bucky balls, are a very new modification of pure carbon and their form resembles soccer balls. They consist out of 20 hexagons and 12 pentagons with a carbon atom in each of edges of this lattice structure. There are only very few applications of this new material class.
How can I come into contact with this material?
Up to now fullerenes are only used in two prominent types of applications which however are not found in Europe. Fullerene nanoparticles are introduced into plastics and other composite materials to improve the products’ properties and a Japanese company is currently producing fullerene-containing cosmetics. Fullerene nanoparticles have excellent radical scavenging properties which is why they were added to an expensive line of anti-ageing cosmetic products resulting in a direct contact of the nanomaterial with the skin. Further possible applications have not yet been put into practice.
Is there any risk from this material to humans and the environment?
So far, there are only a few reliable studies on their health effects on humans or animals. Several studies investigated the distribution of fullerenes in the body and clearly demonstrated that these very small particles (only 0.7 nm in diameter) can penetrate tissue barriers. This fact is only true for individually occurring fullerene nanoparticles which normally exist in an agglomerated state thus reducing the penetration potential of tissue barriers significantly.
Conclusion
Fullerenes are not produced in high quantities and the actual amounts used in everyday products are so small that any possible negative effects can be ruled out. The situation might change however if new products are put on the market that contain considerable amounts of fullerene nanoparticles.
Properties and Applications
Fullerenes (singular: Fullerene) are ball-shaped molecules build from carbon atoms. The so-called Buckminsterfullerenes C60, or buckyballs, are the currently most adequately investigated molecules of that type. These fascinating molecules were named after Richard Buckminster Fuller (1895-1983), an architect who on the occasion of the Expo 1967 designed a dome structure of pentagonal and hexagonal cells (the so-called geodesic dome). The architecture of Fuller’s geodesic dome is very similar to that of the fullerene molecules.
The C60 molecule, for example, is 0,7nm in diameter and, just like a soccer ball, consists of 20 six-membered and 12 five-membered rings, which is why it is often referred to as “soccer ball molecule”. Comparing the dimensions of one such molecule with those of the Earth, one finds, so to speak, that the fullerene-soccer ball relation corresponds to the relation between a soccer ball and the Earth. Fullerenes, just like diamonds or graphite, are carbon modifications. Their very low density (1,68g/cm³) as compared to graphite (2,1-2,3g/cm³) or even diamond (3,51g/cm³) is due to their having the shape of a hollow ball. Fullerenes, as against graphite, are not electrically conductive.
C60 forms yellow crystals but turns to a deep wine red when dissolved in organic solvents (for example toluene). Fullerenes, thus, are the only allotropic carbon modifications that are soluble in organic solvents. The fullerene cage is destroyed by UV radiation, particularly in the presence of O2. The C60 fullerenes are the smallest ones within the large number of allotropic fullerenes. The next larger fullerene (C70), for example, has the structure of a rugby ball. Other Cn molecules (n = 74, 76, 78, 80, 84, 90, 94) have been isolated, and C240 and C540 are assumed to exist in addition.
Fullerenes are currently applied in cosmetics and sports goods industries. Since C60 molecules have a high electron affinity (radical scavengers) due to which they are supposed to absorb many free radicals (reactive molecular species) that are responsible for aging of the skin, they are used, for example, in anti-aging creams. The sports goods industry makes use of fullerenes mainly in golf clubs, badminton and tennis rackets. The C60 molecules are integrated into shafts and frames to obtain very thin-walled, lightweight robust carbon structures.
Since fullerenes are non-biodegradable molecules whose toxicity has not been studied very well so far, companies such as CIBA (since 1st April 2009 part of BASF SE) and Novartis presently refrain from applying them.
Fullerenes are not self-inflammable. As a mixture with air (dust) fullerenes are flammable under the influence of an ignition source (dust explosion). The behaviour in a dust explosion is similar to that of other, carbon-based materials.
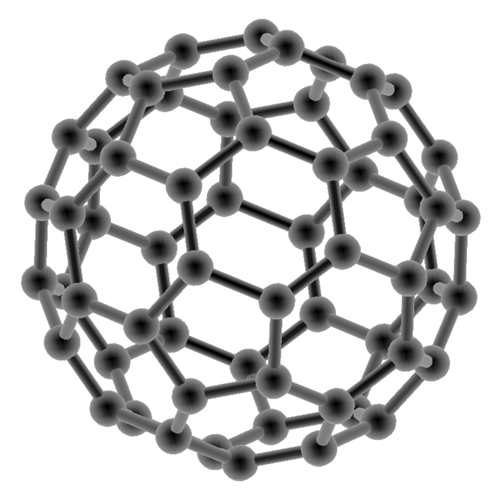
Buckminsterfullerene © www.biocrawler.com
Natural Occurrence and Production
Fullerenes occur naturally in different stones.It is assumed that they have been synthesized by man accidentally in early times and were deposited as soot on the walls of caves. The possibility of the existence of fullerenes was predicted in 1970 by Japanese chemist Eiji Osawa on the basis of calculations. Davidson and Haymet confirmed in 1981 and 1985, the calculations of Osawa. In 1985 Curl, Kroto and Smalley produced for the first time a fullerene, namely C60, in very small quantities via vaporizing graphite in the laser beam , and were awarded the Nobel Prize in Chemistry in 1996 . In 1990, Buckminsterfullerenes were synthesized in a larger scale for the first time by Kraetschmer and Huffmann . Fullerenes are made by vaporizing graphite in the arc or with an electric current in a low-pressure helium or argon atmosphere.
There are only a few applications for this class of materials, so human exposure to fullerenes is very low. Surface-functionalized fullerene are not considered in this evaluation.
General Hazards
Fullerenes can be produced in low amount accidently during combustion-derived processes and thus be released into the air. However, there are only a few applications where fullerenes were used, therefore the exposure of humans and environment is rather low. For this reason, epidemiological data on fullerenes are still not available.
Studies on Living Organisms – in vivo
Independent of inhaled or instilled non-functionalized fullerenes in rats, the particles induced only a transient inflammation reaction in the lung. Low doses of ingested fullerenes have the same transient effect in the liver and lung, but not in the gastro-intestinal tract .
A pretreatment with fullerenes prevented a poisoning reaction in the liver of the test animals with carbon tetrachloride due to the ability of fullerenes to bind free radicals .
The solubility and biological effects are changed drastically through functionalization of the surface of C60, for example via hydroxyl groups or other functional groups. Depending on the modification, the suspension procedure or the application of fullerenes, different toxicological outcome are observed, which cannot always be compared directly.
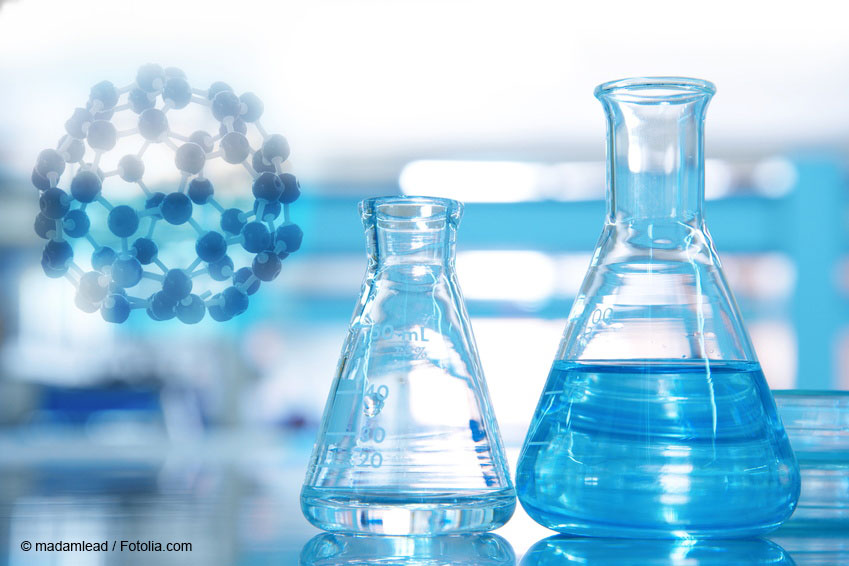
Fullerene with flask © madamlead / Fotolia.com
Studies Outside the Body – in vitro
Fullerenes are difficult to suspend in water due to their chemical properties. They form stable aggregates up to 500 nm in diameter, yet are small enough to be taken up by lung cells.
The toxicity of fullerenes is very controversially discussed. The diverging findings and opinions on the toxicity of C60 result from the different ways of preparing the suspensions needed for the subsequent in vitro testing. If fullerenes are suspended in solvents like Tetrahydrofuran (THF) and thereafter transferred into water, active THF by-products like tetrahydrofuran peroxides are generated accidentally and mainly responsible for most of the acute toxic effects. These effects can be eliminated by removing the by-products through additional washing steps. This finding is confirmed in several studies .
Pure fullerenes, without any solvent or solvent residues do not induce any adverse effects in lung or skin cells.
Fullerenes consist exclusively of carbon, which is common in all living beings and the air. Therefore, a specific detection of these nanomaterials in the environment is very difficult and currently only few data are available on the quantities of fullerenes found in the environment.
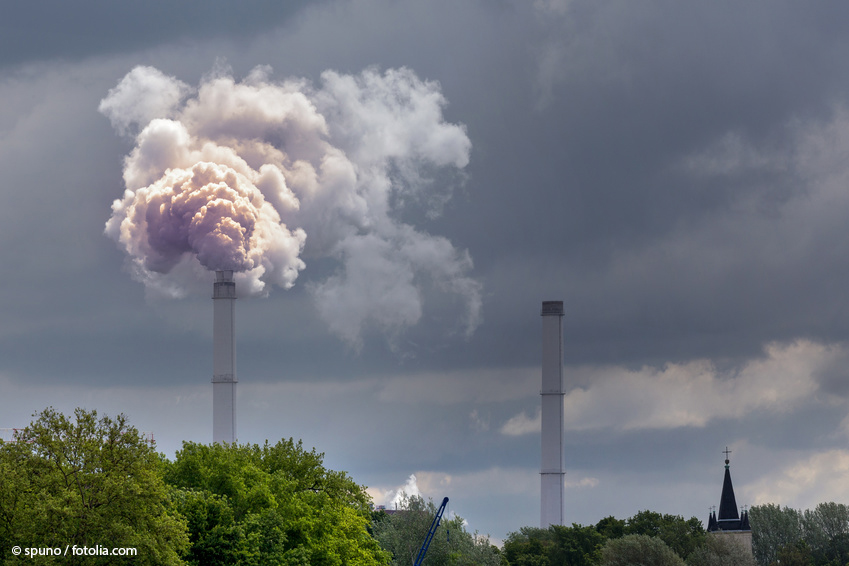
smoke from industrial chimney © spuno / fotolia.com
In addition to production sites, combustion products (soot) from candles, motors, aircraft turbines and the handling of these nanomaterials in the laboratory are sources of fullerenes . Likewise, Fullerenes are also naturally generated by lightning or meteorite impacts. After fabrication, they are released into the air and then transported via the rain into soil or surface waters. Fullerols, which are fullerenes carrying hydroxyl groups on their surface, can be degraded by certain fungi due to their altered surface; this is not possible for fullerenes .
Few studies describe the detection of fullerenes in environmental samples. Traces of fullerene nanomaterials have been found in aerosols of the Mediterranean, in sand from Saudi Arabia, in river sediment from Spain, in waters and sediments from Barcelona and Brazil as well as in some surface waters from Taiwan . However, these small amounts of fullerene nanomaterials do not pose any risk to the respective environmental organisms (see section Uptake & risk for environmental organisms)
In industrial areas however, a tenfold quantity of fullerenes compared to the unspoiled nature was detected, indicating a probable unintentional release by combustion processes .
Although several measurement methods have already been developed for the detection of actual amounts of fullerenes present in the environment, few studies are available. Therefore, computer models were used for predicting environmental concentrations . The expected environmental concentrations (PEC) are calculated from production quantities, predicting a future accumulation of fullerenes in landfills and sewage treatment plants . Subsequently, fullerene nanomaterials originating from sewage treatment plants can either reach surface waters or end up via the sludge in a waste incineration plant.
After evaluating various toxicological studies, the calculated risk quotient for fullerenes was below 1, meaning that the present (theoretical) environmental concentrations of fullerenes do not present any risks for environmental organisms. However, these theoretically calculated values need confirmation in the future by experimentally collected measured values, or adapted to increasing production quantities, respectively.
If and how fullerenes cross the air-blood barrier has not yet been finally clarified. Fullerenes are added to cosmetics and skin care products. However, they specifically penetrate only into the upper skin layers and not into the deeper layers. Surface-modified fullerenes are not considered in the following.
Uptake via the Lung
For example, fullerenes generated during combustion processes can be inhaled as an aerosol similar to other natural or synthetic nanoparticles. However, the amounts of fullerenes unintentionally generated during combustion are very low . In addition to individual free fullerenes, nanoscale and up to 1-micrometer-sized fullerene aggregates can also be taken up into the lungs . The efficiency of the lung self-cleaning process appears to be species-specific, as significant differences were observed between mouse and rat . Instillation experiments of fullerenes in rats showed a mild, transient inflammatory response but no genotoxicity .
Based on the current knowledge of other carbon-based nanomaterials, it is not excluded that fullerenes may cross the air-blood barrier, but only in very low amounts, (normally below the detection limit of the current analytical possibilities) .
Uptake via the Skin
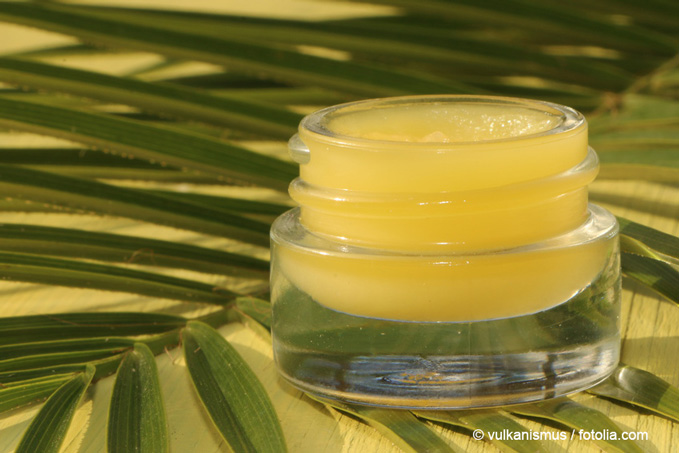
skin care cream © vulkanismus / Fotolia.com
An example for the application of surface-modified fullerenes in cosmetics is a so-called anti-aging cream from a Japanese company. This cream contains lipophilic particles, which only penetrate into the upper skin, but not into the deeper lying skin. (see also tissue barrier – Nanoparticles and the skin) . No reliable results are available on their anti-aging efficiency.
Studies on human skin cell lines showed that pure fullerene (free of solvents like Tetrahydrofuran and solvent residues) are not inducing adverse effects. Novel suspension procedures or chemical modifications of fullerenes can improve the dispersability in an aqueous environment. Generally these modifications do not provoke an additional toxicological risk, however this has to be proved case by case .
Uptake via the Gastro-Intestinal Tract
In a rat study could be shown, that after ingestion of low amount of pure fullerene only a slight increase of DNA oxidation in liver and lung, but not in the GI tract, could be observed. This oxidative stress looks like to be a transient effect, since the level of oxidation normalized after a few days to base line level .
The large variety of fullerenes does not allow any general or comparative statements on their toxicity. Many organisms internalise fullerenes into the body. The fullerene production process, in particular the use of catalysts, and the use of different solvents often influence the toxicity since these substances can also have toxic effects. This makes it difficult to clearly attribute toxic effects to pure fullerenes.
Until 2010, the solvent tetrahydrofuran (THF) has been used in many studies to disperse fullerenes in aqueous media used for biological tests. Such suspensions containing THF-dissolved fullerenes exerted toxic effects on environmental organisms, but toxicity was mainly due to degradation products of the THF in aqueous solutions [zotpressInText item="{4274171:QJ4AFVKC},{4274171:WBZLGK7N},{4274171:A4VDB29R},{4274171:MUNILG3T}"].S olutions with fullerene nanomaterials without degradation products did not cause any negative effects, which is why since 2010 the use of solvents is avoided and alternatives such as stirring or ultrasonic treatment are applied .
Fish take up fullerenes from the water and exert species-specific alterations such as changed fat composition, gill structure, mortality and developmental disorders. An stimulation of stress genes in zebrafish embryos as well as in immune reaction in fathead minnow embryos by fullerenes could also be observed, with light having an intensifying effect .
In mussel blood cells, fullerenes triggered inflammation but were not acutely toxic. In contrast, low concentrations of fullerene nanomaterials also had a toxic effect on oyster embryos, adult oysters, and isolated liver cells. However, toluene was used as the solvent, which could form similar degradation products as THF .
In the case of water fleas, high doses of fullerenes in the water cause increased movement activity and an increased residence of the animals on the water surface. The water fleas were not poisoned in presence of fullerenes, but showed a clear escape behaviour. In a further study, a reduced swimming capacity of the animals was observed. In general, water fleas actively take up the fullerenes from the water into the intestine. The fullerenes do not, however, pass into the body tissue and are presumably excreted completely .However, all observed behavioural changes could be an important indication of a disturbance of the ecosystem. In the case of mosquito larvae, developmental retardation caused by fullerenes has been reported .
High doses of fullerenes in the soil cause reduced fertility in adult earthworms. In addition, damage to the skin and the underlying muscles was observed, but fullerenes do not cause mortality of the animals. On the other hand, younger earthworms were found to be more sensitive to fullerenes. They show slowed growth and have a shorter lifespan. Threadworms reacted in a similar way. They show reduced viability, lower body size and fertility .
In crops such as zucchini and tomatoes, an increased uptake of pesticides after treatment with fullerenes has been observed. Presumably, the pesticides bind to the fullerene nanomaterials and are subsequently co-transported into the plant. Bitter melon plants showed an increase in biomass, water content and fruit number, length and weight under the influence of fullerol. In water plants and algae, on the other hand, fullerenes cause a reduced growth .
Fullerenes also have antibacterial properties. This means that fullerenes can reduce the growth of certain bacteria .
Fullerenes generally have the property of binding other substances, e.g. chemicals. This can affect the availability and toxicity of these chemicals in the environment. Depending on the nature of the chemical, both attenuation and amplification of the toxic effect in algae and water fleas can be observed . Therefore, not only the toxicity of fullerenes alone but also their interaction with other substances present in the environment need consideration.
Concluding, the toxicity of the fullerenes is currently difficult to assess due to their diversity. However, very low doses, as are currently to be expected in the environment, do not pose any threat to environmental organisms. Many other factors, e.g. the role of catalyst residues and impurities, have to be considered.
Pure fullerenes stick together and can be taken up by different types of cells. Once in the bloodstream, fullerenes can spread throughout the body. Surface modified fullerenes were not evaluated.
Behaviour in the body
In rats given a low dose of 0.64 mg/kg of unmodified fullerenes via the diet, mild stress symptoms in the liver and lungs were observed. This is an indirect indication that fullerenes may reach other organs via gastrointestinal absorption . However, direct evidence is not given up to day.
Intravenous Administration and Distribution in the Body
In order to investigate the distribution of the particles in the body via the bloodstream, radioactively labelled, unmodified fullerenes were injected into rats. The fullerenes administered were detected in the liver, lungs, placenta and fetus. Fullerenes were also passed on to the offspring via breast milk . However, the dose of 0.3 mg 14C labelled fullerenes per kg body weight administered in the experiment is high and would not occur in reality. Nevertheless, this experimental approach confirms that not all biological barriers are an obstacle to such tiny nanoparticles as fullerenes (0.7 nm). Similar results have also been found in human placentas .
Behaviour of Uptake in somatic cells
A large number of cells such as macrophages or epithelial cells are able to take up agglomerates of unmodified fullerenes of up to a few micrometers. However, carbon-based nanomaterials can only be detected with great technical effort in tissue or cells .Once in the cell, the particles can be detected in different organelles such as lysosomes, endosomes or in the cytosol. Here they induce, if at all, only mild temporary inflammatory reactions .
Although they consist solely of a carbon framework, there are varieties of different fullerenes, which are mainly constituted by modifications of the particle surface. These modifications affect the properties of the fullerenes and thus also their environmental behaviour, so that no generally valid statements can be made e.g. regarding their mobility.
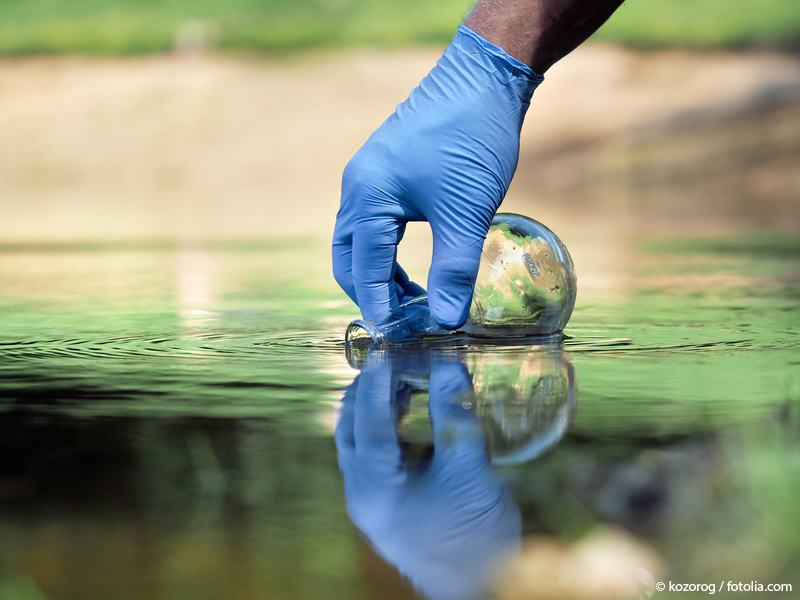
Water sampling © kozorog / fotolia.com
In water or aqueous media, unmodified fullerenes are only poorly dispersed, which can be improved by a change in the surface structure.
Fullerene nanoparticles are rarely found individually, but mostly agglomerated, i.e. in complexes of several fullerenes. The salt concentration, the nature of the salt and the pH value of the water have a decisive influence on the strength of the complex formation [1-3]. Natural organic compounds also influence the agglomeration process and prevent the assembly of the fullerenes as well as their light-dependent transformation .
Irradiation with UV light (a portion of sunlight) can lead to chemical changes on the surface of the fullerenes, thus affecting the fate and transport of the fullerenes in water bodies. Fullerols, which are fullerenes occupied by hydroxyl groups, can even be dissolved by very long exposure to UV light. Due to their poor dispersibility in water, fullerenes preferentially deposit on the bottom of waters. From there, they can be re-distributed partly in the water by binding to naturally occurring particles .
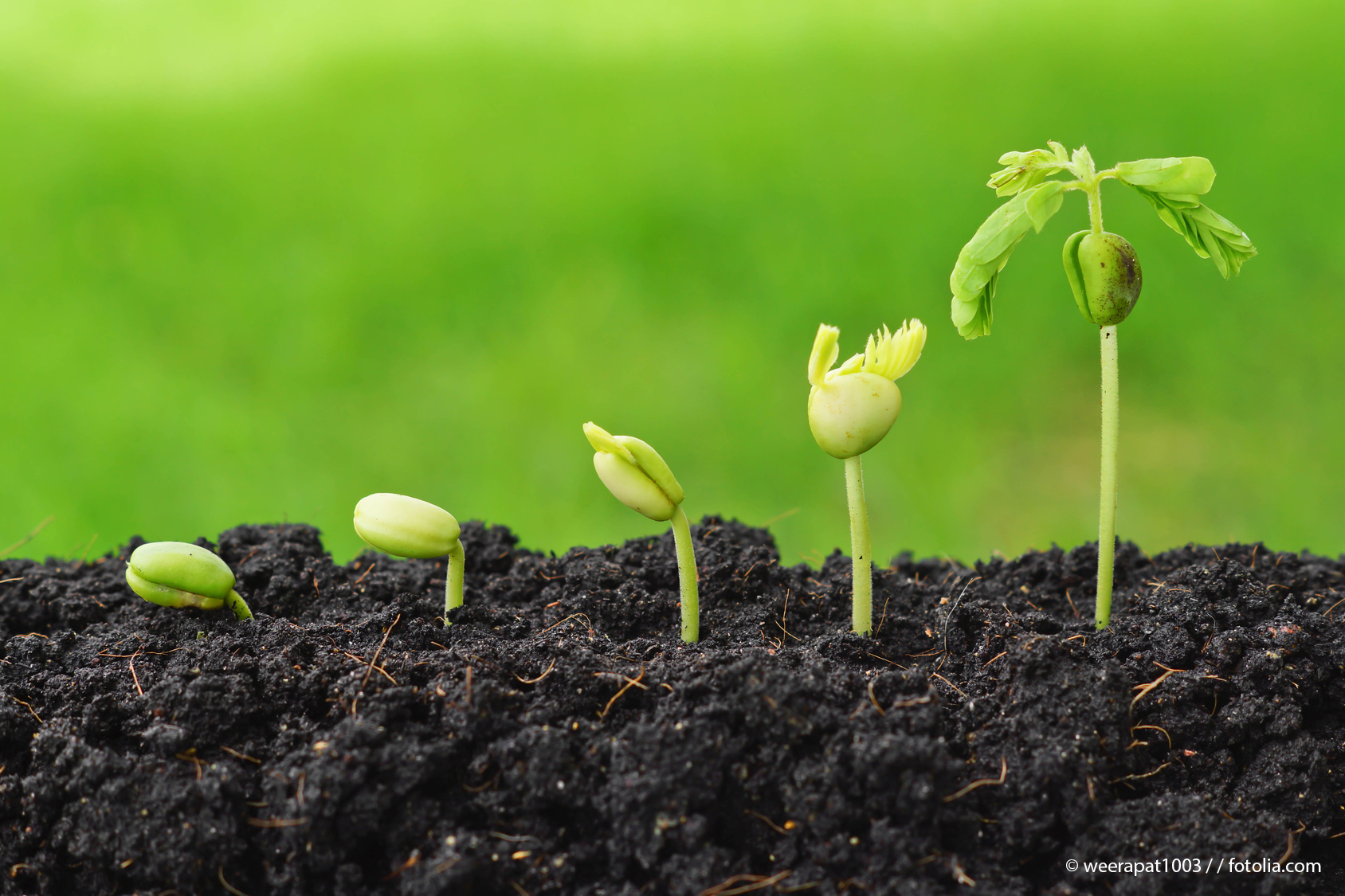
plant germination sequence © weerapat1003 / fotolia.com
The composition of soils has a major influence on the distribution of the fullerenes, in particular the salt content, the pH value, the temperature and the moisture content of the soil. The fullerene nanomaterials are predominantly present as agglomerates, meaning they are less mobile, but able to bind and accumulate more contaminants present in the soil. Depending on their surface structure, the fullerenes can also form a strong bond with the soil components [2,9,13-16].
In general, fullerenes are resistant to decomposition processes. They remain therefore in the environment for a long time and can enter e.g. plants. Certain fungi can degrade fullerols due to their altered surface .
In summary, fullerenes disperse well or poorly in water, which has a decisive influence on their behaviour in water bodies. In addition, they often are not present individually, but rather are connected to agglomerates. These factors make it difficult to compare the different fullerenes and hence general statements on fullerene environmental behaviour are difficult to achieve.