The use of gold and gold nanoparticles can be traced back many centuries: the mixing of gold metal vapours into molten glass turned the colour of the glass into a permanent purple red due to finely distributed gold nanoparticles. Such glass for example was used in jewellery and in many stained glass windows in churches or cathedrals. Today, gold nanoparticles have many more applications: in pregnancy test strips, in cancer diagnostics and cancer therapy (e.g. for breast cancer) and in the binding of atmospheric mercury emissions. Nanoscaled gold particles also play an increasing role in electronics, for example as interconnection between carbon nanotubes in touch-screens.
How can I come in contact with this material?
For centuries the most common way to directly get into contact with gold was and still is through wearing of gold jewellery or via tooth fillings. And it is possible that the abrasion of such gold surfaces may consist of nanoparticles. Some cultures are using gold dust or gold flakes to decorate food (e.g. the schnapps “Danziger Goldwasser”) and in some Asian countries, edible gold is found in fruit jelly snacks or even in coffee. In Europe, textiles made from threads coated with gold nanoparticles are currently in high demand for the fashion industry.
Is there any risk from this material to humans and the environment?
In general, gold has a high biocompatibility and shows only little toxicity. Given that gold jewellery has been worn by people for centuries, it is possible to conclude that human skin has a high tolerance for this metal. If gold nanoparticles are swallowed, they will almost completely be excreted from the human body. For the few cases with a negative reaction to gold, the effects could be attributed to a particular coating material (CTAB = cetyl trimethyl ammonium bromide). The size of the gold nanoparticles plays a big role when looking at possible effects on humans since nanoparticles smaller than 5 nanometers can cause potential adverse health effects. In the meanwhile, experimental studies could confirm that even specifically coated gold nanoparticles were not able to cross the placental barrier.
Conclusion
The long history of mankind with precious metals such as gold has shown that humans have always had regular contact with this material and will still do so in the future. Overall, gold nanoparticles are considered to have a low biological impact.
Properties and Applications
Many people associate with gold prosperity in our time, expensive jewelry and a solid investment. But now the use of small particles of this precious metal, down to the nanoscale, makes it even possible that gold nanoparticles could contribute to solving some critical global problems of today and the near future, because these tiny gold particles have a variety of unique properties that lead to materials covering a wide range of options. Moreover they are still very cost effective, very different from the usual thinking of this expensive precious metal that reaches continuously new record nominal highs.
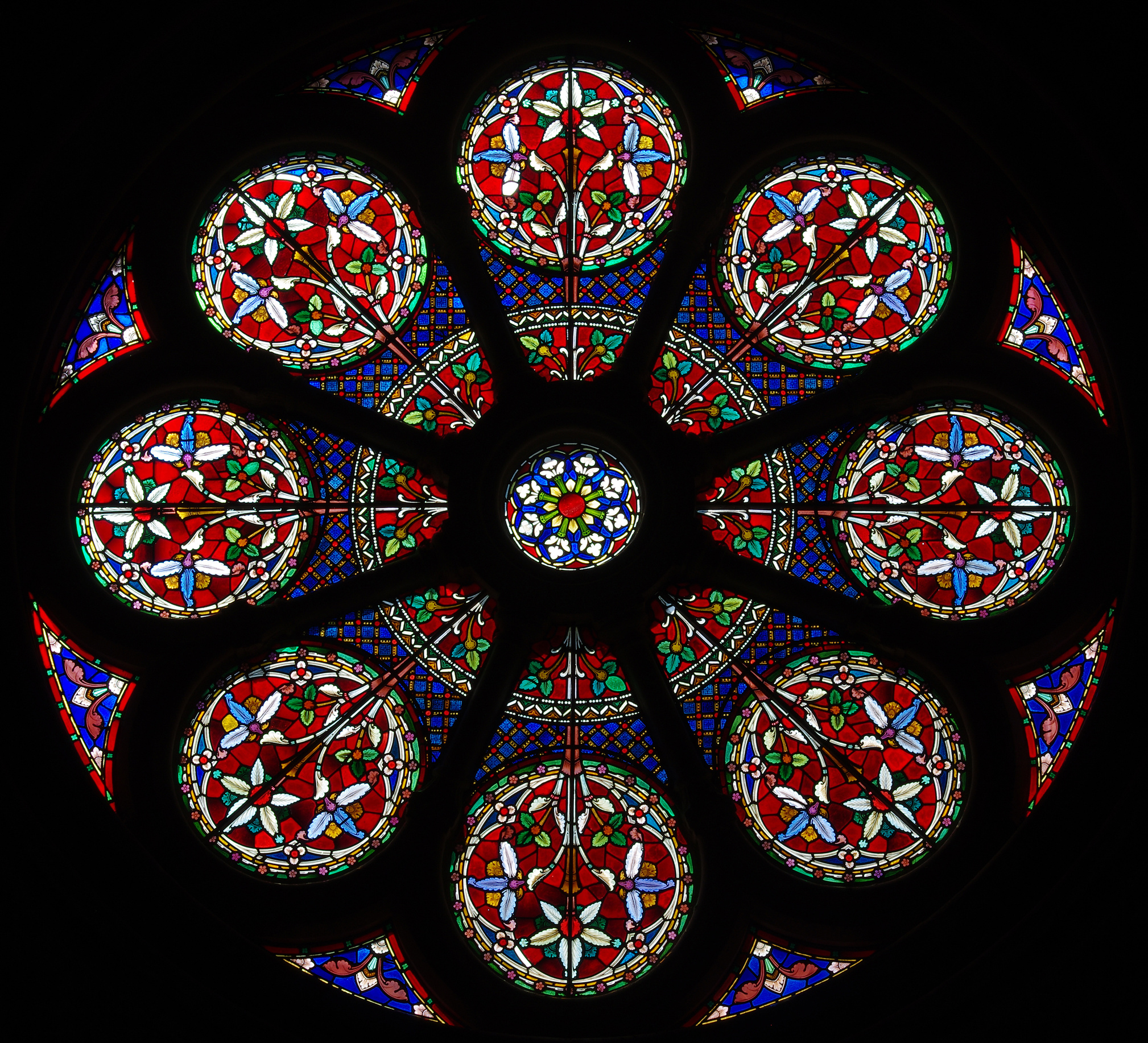
stained glass window in church @ Howgill / fotolia com
These gold nanoparticles have been used for centuries, albeit unconsciously. Roman artisans took advantage of the effect, which was created when they added gold chloride into molten glass: fine gold particles were created, the glass coloring purple-red. Today we know that these particles are nanoscaled. In many cathedrals throughout Europe such colored glass windows can be found.
The earliest medical applications of gold went up to the year 2500 BC. The Chinese used this precious metal to achieve a healing effect. The full potential in biomedical applications, however, revealed nanotechnology. Tumor-targeting techniques that exploit the existing biocompatibility of gold, are being developed as well as other simple to implement, cost effective and reliable diagnostic tests for rapid detection of prostate cancer or other tumors.
Currently, tumor-targeting drug delivery systems with gold nanoparticles are in Phase 1 and Phase 2 of clinical testing. They showed promising results so far. In the field of diagnostics, the precious metal convinces by its testimony reliability and sensitive power with minimal material usage. Thus rapid tests, such as for pregnancy or for Salmonella, E.coli and Campylobacter bacteria, are unimaginable without gold nanoparticles. Likewise, it is already possible to measure by means of functionalised colloidal gold nanoparticles the occurrence of HIV disease and its progress in the body by appropriate rapid diagnostic tests. Similarly, the commercialisation of early prostate cancer diagnostics seems to be possible in the near future by the use of a system, based on gold nanoparticles rapid test methods.
However, the applications of nano gold are not only limited to the medical sector. The precious metal in its nanoscaled form may also contribute to the more efficient and “greener” production of some of today's chemical intermediates used in the production of our everyday commodities and food products. Also the tiny gold particles can be used in air and water purification, for example via the efficient binding of mercury emissions into the atmosphere. Attempts were made to produce cheap and efficient fuel cells with (nano-)gold, a major clean-energy technology of the near future.
One of the largest and still growing industries, the information and communication technology (ICT), can also hardly refrain from the use of gold. Here it is used for the development of conductive nanoparticle inks, for example, to print on plastics for electronic applications, because it has high durability and excellent resistance. Further investigations on touch-sensitive displays with gold nanoparticles show enhanced values, as well as these particles can cause a further improvement of flash memory devices (e.g. USB flash drives or SSD hard drives). In particular, the current standard conductive material for touch screens indium tin oxide (ITO), could be replaced by the combination of nanoscale gold and carbon nanotubes (CNTs) in a transparent layer. Other possible applications are in the area of solar cells.
The numerous applications of gold nanoparticles are mainly based on the unique properties of these tiny particles. They show very high biocompatibility and are thus non-toxic, which makes gold interesting for biomedical applications. Due to the so-called surface plasmon resonance effect the colour of the particles changes when targets such as hormones or antigens are being bound. Using the pregnancy test as an example: once a specifically modified gold nanoparticle binds to a characteristic hormone that is only produced during the early stages of a pregnancy and that can be detected in a urine or blood sample, the colour change occurs and thus the pregnancy can be detected.
Gold is not self-inflammable as nanometer-sized powder. Also as a mixture with air (dust) under the influence of an ignition source, gold is not inflammable, so there is no possibility of a dust explosion.
Natural resources and manufacturing
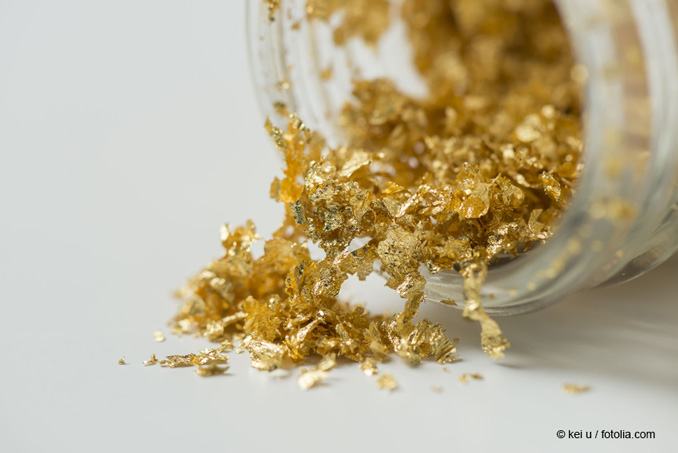
gold flakes © kei u / fotolia.com
Gold occurs on earth in the primary raw materials as gold-bearing rock (gold ore) and in secondary deposits as fine metal. The world's annual production is currently about 2.600 tons, about one hundred times more than in the 19th Century. Significant amounts of gold are produced during the refining of other metals such as copper, nickel or other metals. So sometimes only these "impurities" lead to an economical exploitation.
Large parts of gold nanoparticles are now obtained via the reduction of gold chloride solution (so-called tetra-chloro-aureate). This process uses different materials as a reducing agent, such as citric acid, oxalic acid, boron hydrides or others. Alternatively, it is also possible to generate such nanoparticles via laser radiation or UV light irradiation. In addition to the reducing agent a stabiliser is added during the process. This and the choice of the process parameters significantly affect the size, shape and morphology of nanoparticles.
Further Information
- Keel, T., Holliday, R., Harper, T. (2010). Gold for good - Gold and nanotechnology in the age of innovation. World Gold Council, January 2010, London, UK.
Gold nanoparticles are taken up by cells. They have been described as both toxic and non-toxic. This difference depends on the size, surface charge and particle coating.
Studies on Living Organisms
Studies in rats, mice, pigs and fish have shown discrepancies of the toxic effects of different gold nanoparticles. Nevertheless, they distribute all over the body and accumulate to some extent in the liver, spleen, lung, brain, kidney, testis and heart . The gold nanoparticles have been found to be not toxic in zebra fish .
As for in vitro experiments the distribution and the effects in whole organisms are strongly dependent on the size of the gold nanoparticles . Only the small gold particles (<10 nm) could be found in the brain of animals after injection of a certain dose. 50, 100 and 250 nm gold particles did not reach the brain . This result could be confirmed by other groups , which clearly demonstrated that after injection or oral administration only the very small particles reached organs like the brain, heart or lungs. Of course after injection the total amount of the administered dose could be found in the body and most of the particles accumulated within the liver. For the large particles more than 99 % were found in the liver, whereas from the smaller particles around 1 % could be found in the brain, heart and uterus .
Studies Outside of Organisms - in vitro
In several studies it has been shown that gold particles are toxic when size is less than 5 nm in diameter and they are non-toxic when larger than 10 nm . The conflicting results regarding the toxicity of gold nanoparticles, thus, reflect mainly the differences in their physico-chemical properties but also the variability in the cell systems and the assays used. Actually, most of these studies are summarized in an overview recently published . Besides the fact that the very small gold particles are most toxic, those with negative surface charge show a toxic effect (<3 nm). The gold nanoparticles can enter the cells via vesicular uptake, but they do not enter other organelles, e.g. the cellular nucleus .
Stable gold particle suspensions in physiological media can only be achieved if the particles are coated . Therefore the effects of the coatings have been investigated as well. The shape of the gold particles was not crucial for their effects as no direct toxicity was found for nanorods of different aspect ratios . The cytotoxicity shown in this study could be clearly related to the chemical substance cetyltrimethylammonium bromide (CTAB) used to stabilize the gold nanorods. The same study demonstrated that serum proteins from the growth media were found to adsorb on the gold nanoparticles, which slightly increased the net size of the nanoparticles and also converted the surface charge to that of the bovine serum albumin. The results show that each of the gold nanorod preparations, regardless of initial surface charge, adopted the charge of serum proteins and were found to enter the cells. This is a well-known effect for metal oxide nanoparticles as well as carbon-based nanoparticles (e.g. carbon nanotubes) .
A more realistic in vitro model, a triple cell co-culture model at the “air-liquid interface”, revealed no adverse effects, not even in an inflammation stimulating environment . Non-functionalized gold particles with a size of 13–20 nm in diameter do not cause acute adverse effects.
Gold nanoparticles of various sizes have been shown to be taken up by cells via different mechanisms but they end all within vesicular structures . The cells totally recover after removal of the gold particles from the supernatant.
So far no data exist on environmental exposure with engineered gold nanoparticles. However, it is known that nanoscale gold can also be produced naturally by the activity of microorganisms. Its occurrence is limited to places and waters, in which gold and gold compounds in rock or earth are present .
Hardly any valid studies are known on absorption via the lungs or skin. If gold is ingested with food, 99.5 % is excreted.
Uptake via the Gastro-Intestinal Tract
After oral uptake more than 99,5 % of the administered particles have been found within the feces, totally independent from the size of the particles, which have been applied. Less than 0,5 % has been translocated into the circulation. The smallest particles (1,4 nm and 1,8 nm) distributed best within the body, and negatively charged particles more than positively ones .
Gold nanoparticles have been investigated in a number of cell lines and organisms in terms of their absorption and toxicity. These studies show that gold particles are taken up into cells and organisms, but are not or only slightly toxic.
Water fleas take up their food by filtering water. If the water contains gold nanoparticles, they are detected within a short time in the intestines of animals. However, they are not absorbed from the intestine into the surrounding tissue. If the fleas are then kept in particle-free water, the particles are transported through the intestine and excreted . Only very high concentrations caused a mortality in water fleas, reproduction and embryo development was not affected by nanoscale gold . However, particles were observed to adhere to the carapaceof the animals, affecting both the swimming behavior and the molting rate.
Gold nanoparticles (3, 10, 50 and 100 nm) were demonstrated to be incorporated into zebrafish embryos, but did not lead to malformations or to increased mortality . Gold nanoparticles with a size of 12 nm can pass through the pores which are also used for the transport of other substances into the zebrafish embryo . The particle transport is carried out passively and concentration-dependent, i.e. the more particles are present in the vicinity of the embryo, the higher is the concentration in the embryo. The uptake of particles is accompanied by a small increase in mortality compared to control, and a likewise small increase in the number of malformations of the developing fish.
In gold-nanoparticle-exposed liver cells of rainbow trout, the formation of reactive oxygen species was observed. However, these had no effects on the viability of the cells. Gold nanoparticles and gold ions in the same concentrations had similar effects on the cells. This shows that probably not the nanoparticulate form, but the element gold causes the observed effects. A simultaneous exposure to dissolved organic materials, as those found in normal surface water, does not change the effect of the nanoparticles .
Blue mussels accumulated gold nanoparticles almost exclusively in their digestive glands. In addition, the particles triggered oxidative stress in the glands, but not in the gill and mantle tissue . These results were confirmed for two different particle sizes (~5 and ~13 nm) . For another shell species, the basket shell, similar findings have been described .
In a study of bacteria, cucumber and lettuce plants 10 nm gold nanoparticles exerted no toxic effects . For the solvent used much stronger effects were observed than for the nanoparticles alone. Gold nanoparticles had a positive influence on the growth of lettuce plants, as an increase in shoot growth was observed after 15 days of exposure . Amine-coated gold nanoparticles adhered to the surface of algal cells, as a consequence a concentration-dependent growth reduction of 20-50 % was observed. However, no particles were incorporated into the algal cells .
Luminescent bacteria treated with nanoscale gold took up the particles and showed a decrease in their luminosity . The change of this parameter was caused by a slowdown of cell metabolism, but not with increased mortality. No influence on bacterial growth behavior was observed for gold nanoparticles coated with polyethylene glycol (PEG) (30 nm) . The number of microorganisms in the soil was reduced by gold nanoparticles, but only a very small extent .
In summary, despite an uptake of nanoscale gold in the cells or the intestines of many organisms, little toxic effects were observed. Comparing the organisms studied, algae reacted the most sensitive to exposure to gold nanoparticles.
Only the very small gold nanoparticles can pass tissue barriers.
Behaviour inside the Body
After injection of gold nanoparticles of different sizes into rats only the very small ones could be found in the brain or other soft tissues . The same result was obtained by using PEGylated gold nanoparticles of the size 10-30 nm. These did not penetrate the placenta within a perfused human placenta system during the 6 hours observation time .
Behaviour of Uptake in somatic cells
Experiments with inhibitors of different uptake mechanisms demonstrated that gold nanoparticles are taken up via different vesicular endocytosis pathways but dependent on the size of the particles .
Dissolved organic matter occurs in all surface waters and influence the behavior of gold nanoparticles in the environment . The results of the existing studies are contradictory.
No stabilization of nano-scale gold (in contrast to many other nano-materials) was observed, as well as an effective prevention of the agglomeration of gold nanoparticles by natural organic compounds. Citrate-and acrylate-stabilized gold nanoparticles were stable under conditions typical for fresh water. At extreme pH values and salt content (such as in sea water), this effect is not observed, however, and there is an agglomeration of particles .