Titanium dioxide nanoparticles are the main component of sunscreens and wall paints. Thus humans generally come into contact with Titanium dioxide nanoparticles via the skin. Accidental ingestion should not be a problem, since titanium dioxide is no longer approved as a food additive. There is no significant evidence for a nano-specific risk.
How can I come into contact with this material?
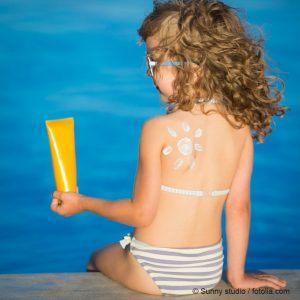
child with sunscreen © Sunny-studio /fotolia.com
By applying sunscreen to your skin you also apply titanium dioxide nanoparticles. These particles however do not pass through healthy or sunburnt skin. Wounds however should be treated with wound ointment instead. Self-cleaning wall paints contain Titanium dioxide nanoparticles which are fixed within the paintwork after drying, thus humans do not get exposed to these particles. In addition, titanium dioxide contained in tiles, flagstones and solar panels are also present in a bound manner. As there are no titanium dioxide nanoparticles released during the day-to-day use it is highly unlikely for these particles to enter the human body.
However, in all major applications the bound titanium dioxide nanoparticles may eventually leach out into the environment, but negative effects for humans or wildlife have shown so far for only very high doses or concentrations. Nevertheless, this requires further research.
Is there any risk from this material to humans and the environment?
Titanium dioxide is toxic in very large concentrations. This means that inhalation of titanium dioxide particles, as for all dusty particles, should be avoided. Damage to the lungs after high doses has been proven in animal experiments. These and other studies have also led to titanium dioxide no longer being permitted as a food colorant (E 171) in the EU as of August 2022; this ban does not apply to drugs or other medical applications.
Conclusion
Titanium dioxide nanoparticles do not enter the human body through intact skin. In other applications than sunscreen the nanoparticles are generally not free to move, because they are firmly bound in a matrix.
By the way…
All grinding work causes dust which always contains a certain amount of nanoparticles regardless of whether the ground material previously did contain any nanoparticle. The amount of particles in the air (dust) can be reduced by wet grinding, which also reduces the generation of nanoparticles.
Properties and Applications
Titanium dioxide (TiO2) has become part of our everyday lives. It is found in various consumer goods and products of daily use such as cosmetics, paints, dyes and varnishes, textiles, paper and plastics, drugs, and even paving stones. 4.68 million tons of titanium dioxide were produced worldwide in 2009 1,5 million tons/year are produced in the European Union. Production was even higher before the financial crisis in 2007 and 2008.The great versatility of titanium dioxide is owing to its various forms and sizes. Titanium dioxides may be used in the form of microscale pigments or as nano-objects. Their crystal structures may vary: Depending on the arrangement of Titanium dioxid atoms, one differentiates between rutile and anatase modifications.
Due to its high diffraction index and strong light scattering and incident-light reflection capability, Titanium dioxid is mostly used as white pigment. It is these properties and a high UV resistance that make Titanium dioxid the standard pigment found in white dispersion paints with high hiding power. Since light scattering does not occur anymore in nanoscale particles, the white titanium dioxide pigments used are almost exclusively rutile modification particles with grain sizes in the micrometer range. These white pigments are not only found in paints and dyes but also in varnishes, plastics, paper, and textiles. Having the label E171, it was listed as a food additive in the EU until it was banned in August 2022, but is still allowed in toothpastes, various other cosmetics and medicines. Titanium dioxid pigments for use in plastics constitute the fastest growing market. It is in particular due to the packaging industry’s strong demand that the consumption of titanium dioxide pigments is on the increase.
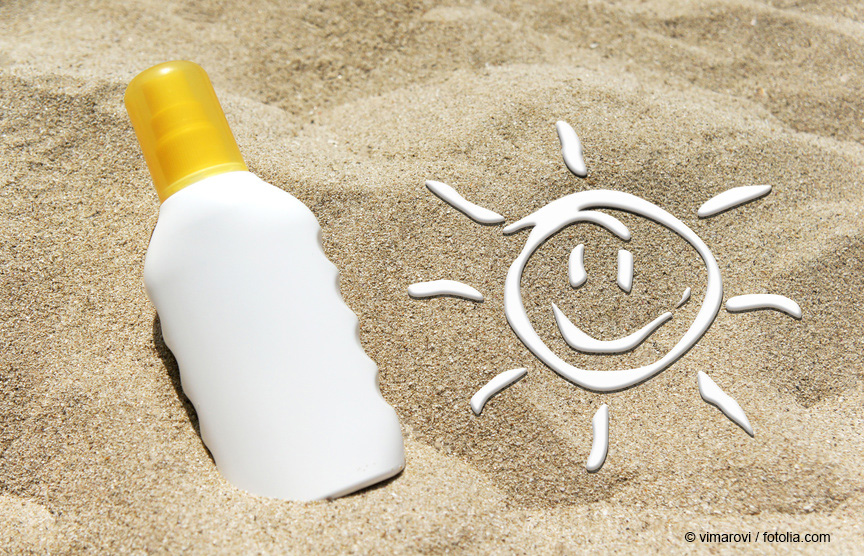
© vimarovi / Fotolia.com
Nanoscale titanium dioxide that is manufactured for specific applications is by approximately a factor of 100 finer than the Titanium dioxid pigments and has other physical properties. The production volume of nanoscale Titanium dioxid amounts to less than 1 percent that of TiO2 pigments. Currently, they are mainly found in suncreams, textile fibers or wood preservatives. For a long time, suncreams have been manufactured adding titanium oxide microparticles that gave the products a pasty, sticky consistency. Leaving a visible film, application of such suncreams was not easy and not pleasing to the skin. Suncreams that contain the transparent nanoscale titanium dioxides can be applied much more easily. In addition, their protective effect against harmful UV radiation is much better.
The German Association for Cosmetic, Toiletry, Perfumery and Detergent (Industrieverband Körperpflege und Waschmittel e.V. - IKW) has been reporting that only nanoscale titanium dioxides are used in sunscreens presently.
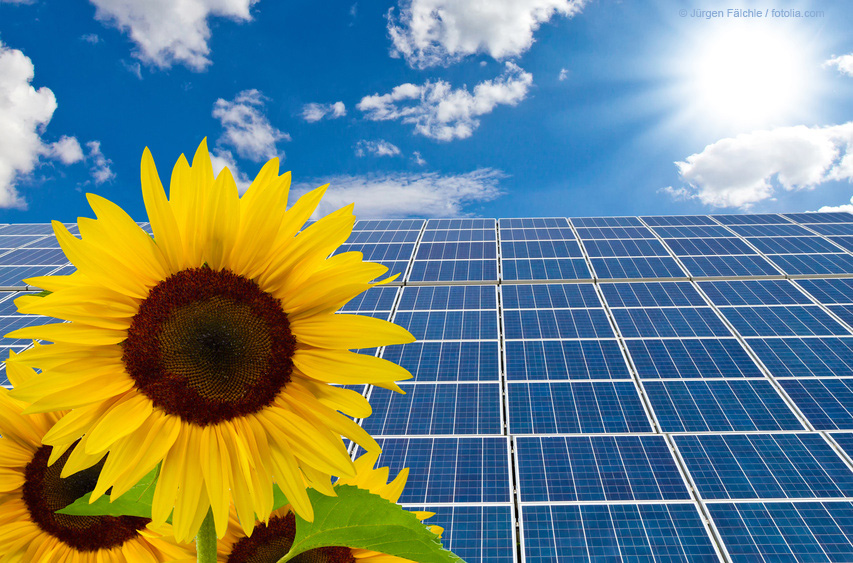
Solar cells © Jürgen Fälchle / Fotolia.com
To achieve better dispersion properties and ensure photostability, these Titanium dioxid particles, moreover, are coated with further materials . The photocatalytic activity, which is another property of Titanium dioxid, is increased considerably through the high surface-to-volume ratio of the nanoparticles as compared to that of microparticles. However, not each of the above modifications can be used for photocatalytic purposes. While, as has been shown above, rutile Titanium dioxid are applied mainly in suncreams, paints, and dyes, anatase modifications are rather suited for photocatalysis. In the presence of UV radiation, anatase Titanium dioxid can form radicals from air or water which can degrade oxidatively organic pollutants. In Japan, paving stones and ceramic tiles are used along traffic routes that can "clean" the air of car exhaust fumes with the help of titanium dioxide. Researchers at Universität Kassel have found a method of interlocking nanoscale Titanium dioxid with dye molecules in such a way that the photocatalytic process can be triggered also by visible light and not exclusively by UV radiation.
Due to the hydrophilic character of titanium dioxide, water forms a closed film on the surface in which pollutants and degradation products can be easily carried away. House paints or tiles containing Titanium dioxide particles thus are self-cleaning and pollutant-degrading. Besides, so-called anti-fog coatings benefit from the hydrophilic properties of nanoscale titanium dioxide. The ultra-thin water film on a glass pane coated with a transparent layer of nanoscale Titanium dioxide impedes the formation of water droplets and, thus, avoids fogging. Nanoscale titanium dioxides are also suited for use in dye-sensitized solar cells (Graetzel cells).
Titandioxid is not self-inflammable as nanometer-sized powder. Also as a mixture with air (dust) under the influence of an ignition source, it is not inflammable, so there is no possibility of a dust explosion.
Natural Occurence and Manufacture
Titanium dioxide mostly occurs together with other types of rock, thus must be separated from these. Ilmenite (FeTiO3) is one of the most well-known minerals. Different methods are used for refinement.
In the European Union, 70 percent of all titanium dioxide are extracted from natural minerals using the sulfate method while the remaining 30 percent are obtained by means of the chloride method. In Germany, both methods are used equally. The sulfate method came under criticism some decades ago for producing dilute sulfuric acid (referred to as dilute acid) which through to the eighties was dumped in the North Sea. North Sea dumping has been forbidden in Germany since 1990. Today, dilute acid is being treated or fed into manufacturing processes. During the chloride method, Titanium dioxide ores react with chloride gas while forming hydrochloric acid. Being much more significant to industry than dilute acid, hydrochloric acid can be recycled into production or be sold.
Further processes are necessary for production of nanoscale Titanium dioxide. The so-called titanium alkoxylates can be hydrolysed and subsequently be treated thermally. The particles’ crystal modification depends on the temperature applied during the process. Moreover, nanoscale titanium oxide particles can be obtained by reacting titanium chloride compounds with ammonia. Under the influence of heat, the titanium oxide hydrate forming during that reaction turns into rutile Titanium dioxide. The aerosol method that was developed by Degussa in the forties for silicon dioxide was applied to titanium dioxide in the fifties. It enables production of nanoscale titanium dioxide from titanium chloride compounds through reaction of the latter with water vapor.
NanoCare Data Sheets
- Titanium Dioxide data sheet No.1 (PDF)
- Titanium Dioxide data sheet No.2 (PDF)
- Titanium Dioxide data sheet No.3 (PDF)
- Titanium Dioxide data sheet No.4 (PDF)
- Titanium Dioxide data sheet No.5 (PDF)
Further information
- PR Web.com (EN) (30.08.2010) . Global Titanium Dioxide Industry Stabilises and Heads for Recovery, TZMI Pressemitteilung.
- Cefig.org (EN): Titanium Dioxide Manufacturers Association (TDMA) (Stand letzter Zugang: Sep 2011).
- Cefig.org (EN): Industry responds to Nano-TiO2 study published in American Association for Cancer Research Journal, Offener Brief des Titanium Dioxide Stewardship Council vom 3. März 2010.
- NanoTrust Dossier No.008en (Dec 2010). Nanotechnology in Cosmetics, NanoTrust, Institute of Technology Assessment (ITA), Vienna Austria.
- Scientific Committee on Consumer Products (SCCP) (19.06.2007). Safety of nanomaterials in cosmetic products.
Titanium dioxide is used in many everyday products. A reassessment of some existing studies led to concerns in 2020. If inhaled in large quantities, titanium dioxide can pose a certain risk of lung cancer. For this reason, its use has been severely restricted. Titanium dioxide was banned in food in 2022.
Everyday Contact
Although epidemiological studies have not yet provided any evidence of an increased risk to workers or consumers, the use of titanium dioxide in food as an additive was banned in the EU in 2022. It must be labelled separately in products for daily use. The reclassification in 2021 is based on experiments with rats and very high doses administered. However, the quantities of titanium dioxide used in the studies are not to be expected in everyday products.
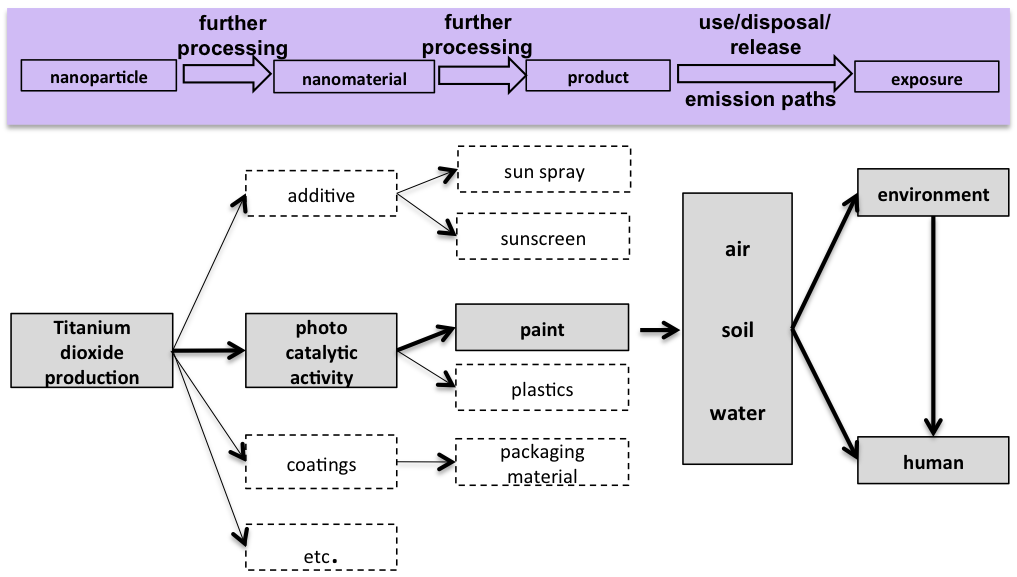
Life cycle and possible paths of titanium dioxide release. © Kuhlbusch et al., UBA-Study
Situation at the Workplace
To date, none of the epidemiological studies have provided evidence of an increased risk of production workers developing lung cancer or other types of cancer. The mortality rate is also not increased .
Workplace exposure studies as part of the NanoCare project showed that mainly particles larger than 450 nm are released during the filling of TiO2 powder. These are therefore not nanoparticles .
Nevertheless, the risk of inhaling dust containing titanium dioxide is highest at the workplace in the processing industry. For this reason, all relevant limit values for so-called "granular biopersistent dust particles" (GBS, see also Granular biopersistent dusts) naturally also apply to titanium dioxide particles regardless of their size.
Products and Consumer
So far, consumers have come into contact with titanium dioxide via all routes of intake. As a UV filter in sunscreens or cosmetics or as a colour pigment in wall paints via the skin, as a food additive via food or also via the lungs during sanding work.
As titanium dioxide has been banned in food since 2022 and is only used to a limited extent in cosmetics by manufacturers, these routes of intake have been significantly reduced, although there is no evidence to date of negative effects from titanium dioxide in these products. Titanium dioxide is still permitted as an excipient in medicines, as there is no substitute for it. A ban in medicines would mean a further shortage of important medicines on the European market (since 2022).
Exposure has decreased significantly after the ban in food and the restrictions in cosmetics. However, for more than 40 years there has been no evidence of any health effects in consumers from the use of titanium dioxide. There have also been no concerns in the workplace due to epidemiological studies, as these have all been negative.
Further Information
- EC (2022): COMMISSION RECOMMENDATION of 10 June 2022 on the definition of nanomaterial. Download: https://eur-lex.europa.eu/legal-content/EN/TXT/PDF/?uri=CELEX:32022H0614(01)&from=EN
Due to the use of titanium dioxide (TiO2) nanoparticles in everyday products, increased exposure in bodies of water (i.e. the aquatic environment) is expected. For example, a washing out of particles from paint or color, which are used outside of buildings and are exposed to wind and rain is conceivable. Likewise, particles from sunscreen-treated skin may reach surface water or wastewater during bathing or showering.
It is not easy to detect TiO2 nanoparticles in the environment. However, titanium dioxide nanoparticles were found to leach from facades painted with TiO2-based paint [1]. The particles run down with the rain water in form of aggregates, and often embedded in the paint components, and reached surface waters.
On examination of sediment samples from a region in China, engineered TiO2 particles were detected by electron microscopy and it was possible to distinguish them from naturally occurring titanium [2]. At the same time, this study showed that the accumulation of Ti in sediments has been going on for decades, since also coarser particles produced earlier are detectable. As the sources of Ti pollution, the introduction of treated and untreated waste water is assumed.
An investigation of effluent from sewage treatment plants [3] showed that a large proportion of titanium particles is removed from the wastewater, however, the very small (<700nm) particles remain in the water, and so again may reach rivers and lakes. The titanium concentrations in the effluent of the treatment plant were 5 to 15µg/l. During the purification process, most of the titanium particles are bound to solids and get into the sludge. The latter, in turn, is either disposed of as landfill or spread on fields as fertilizer, so that the coarser titanium particles are more likely to get into the soil.
Because the TiO2 concentrations in the environment are so low, both the development of measurement methods [4,5] and the simulation of exposure to titanium dioxide nanoparticles in the environment [6] are currently in the focus of research and development.
By means of computer programs, it has been attempted to simulate the probable behavior of titanium dioxide nanoparticles in the environment. Therefore, they most likely occur in natural surface waters and their sediments and in sewage sludge and soils on which sewage sludge was disposed [7,8]. Comparing these predicted environmental concentrations (PEC values) with concentrations not just hazardous for environmental organisms, (PNEC value), it is apparent that currently; particularly TiO2 nanoparticles in discharge from sewage treatment plants may pose an environmental risk. However, for surface water, soil, and air no risk is expected at present. The figure explains in more detail how such a risk is calculated.
Risk Ratios for TiO2 in different Regions |
|||
Environmental compartment | Europe | USA | Switzerland |
Surface waters | 0,015 | 0,002 | 0,02 |
STP effluent | 3,5 | 1,8 | 4,3 |
Air | <0,0005 | <0,0005 | <0,0005 |
The risk ratio is calculated from the predicted environmental concentrations (PEC) divided by the concentrations that have no effects on environmental organisms (PNEC). If the risk ratio is less than 1, there is no immediate risk to the environment, whereas at levels above 1, there is a risk and further investigations must be carried out [7].
Another computer simulation assumes that in the future, the amount of produced TiO2 will continue to rise and that the proportion of nanoscale TiO2 also further increases [9]. From this, it is concluded that the environmental concentrations will increase in the future.
Generally, there are still large gaps in knowledge in this area which are mainly due to inadequate methods of measurement and, hence, accurate knowledge of the environmental concentrations is missing. Further, data are lacking on accurate amounts of substance, as well as on the behavior and distribution in the three environmental compartments water, soil, and air.
Literature
- Kaegi, R et al. (2008), Environ Pollut, 156(2): 233-239.
- Luo, Z et al. (2011), J Environ Monit, 13(4): 1046-1052.
- Kiser, MA et al. (2009), Environ Sci Technol, 43(17): 6757-6763.
- Tiede, K et al. (2009), Water Res, 43(13): 3335-3343.
- Contado, C et al. (2008), Anal Chem, 80(19): 7594-7608.
- Gottschalk, F et al. (2010), Environ Modell Softw, 25(3): 320-332.
- Gottschalk, F et al. (2009), Environ Sci Technol, 43(24): 9216-9222.
- Mueller, NC et al. (2008), Environ Sci Technol, 42(12): 4447-4453.
- Robichaud, CO et al. (2009), Environ Sci Technol, 43(12): 4227-4233.
Titanium dioxide can be taken up via the lungs or the gastrointestinal tract. Due to its many layers, the skin is a good barrier for particles. The addition of titanium dioxide in nano form to food or food packaging was never permitted in Germany. Since August 2022, titanium dioxide (E171) in any form and size has been banned as a food additive.
Uptake via the Lung
As part of the NanoCare project, in vitro tests were carried out with human lung cells. Different variants of titanium dioxide only caused loss of vitality after administration of very high doses (≥ 50 µg/cm2). Cells exposed to titanium dioxide particles for 2 and 4 hours in the so-called "Karlsruhe exposure system" to simulate dust formation showed no loss of vitality at any of the concentrations used and there were no signs of acute cytotoxicity .
A 5-day inhalation study conducted in the NanoCare project showed that inhaled titanium dioxide particles are deposited as agglomerates in the lungs, are then taken up by macrophages and are also deposited in lung-associated lymph nodes when high doses are administered (50 mg/m3). In contrast, nanoparticles were not detected in the liver, kidneys, spleen or basal brain with attached olfactory bulb in these studies [.
Instillation studies with low (0.6 mg/lung) and high doses (4.8 mg/lung) also carried out as part of the NanoCare project showed a slight dose-dependent increase in the number of macrophages in the lungs. These cells are important for immune defence and serve to remove foreign substances, such as particles, by phagocytosis.
Further instillation studies have shown that titanium dioxide particles can be deposited in the lungs at low doses and can also be transported to the liver and kidneys. The low doses of titanium dioxide had no negative effects on the lungs, but led to a temporary change in the metabolites in the liver and kidneys. In contrast, a strong aggregation and deposition of particles in the lungs was observed at high doses. This led to strong inflammatory reactions. However, no further transport of titanium dioxide particles into the liver and kidneys was detected. The macrophages could no longer perform phagocytosis, so the lungs were overloaded with particles .
Kobayashi and colleagues also describe in their studies that particles in the lungs can cause short-term effects (24 h) However, the inflammatory reactions observed had subsided again after approx. 1 month .
In 2021, the ECHA reclassified titanium dioxide on the basis of several studies with high-dose experiments on rats. Everyday products containing titanium dioxide, regardless of size, must be labelled according to EUH212 as follows: "Warning! Hazardous respirable dust may be generated during use. Do not inhale dust". However, on 23 November 2022, the European Court of Justice annulled the Commission Regulation from 2019 insofar as it concerns the harmonised classification and labelling of titanium dioxide in certain powder forms as a carcinogenic substance if inhaled. In its judgement, the General Court states that the Commission had made a serious mistake in assessing the reliability and recognition of the studies. The classification was based on this erroneous assessment.
Uptake via the Skin
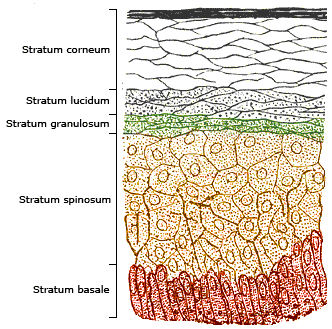
Human Skin Layer © Wikipedia.de
Titanium dioxide particles are not taken up into the body via the skin. This was investigated and confirmed in the EU project NanoDerm for nano-titanium dioxide particles as a component of cosmetics . Further studies confirm this by investigating pig skin, which is very similar to that of humans . Another very good overview on the safety of titanium dioxide particles in sunscreens comes from France, which also found no concerns for the use of titanium dioxide in sunscreens from all the studies consulted .
In order to obtain good dispersion properties of the sun cream, to ensure photostability and to prevent the formation of harmful so-called reactive oxygen species (ROS), the titanium dioxide particles are also coated (see also NanoDerm final report, 2007).
The question remains as to how injured, inflamed or particularly sensitive skin, such as skin damaged by sunburn, reacts? A study from 2011 was able to show that titanium dioxide and zinc oxide nanoparticles are also unable to penetrate UVB-damaged skin (sunburn) .
The particles remain in the upper layers of the epidermis (see the article under "Basics Basic Information - How can innovative materials (e.g.nanomaterials) enter the body or the environment?")
Sun creams with titanium dioxide and zinc oxide nanoparticles offer efficient protection against skin damage caused by ultraviolet light (UVB).
Uptake via the Gastro-Intestinal Tract
Orally ingested insoluble mineral particles are usually excreted in the faeces. To date, however, only a few analyses have been carried out on this topic.
Studies with treated human intestinal cells (20 and 80 µg/cm2) certainly showed toxic effects after 24 hours of treatment. However, DNA damage was not observed . Titanium dioxide was authorised as a food additive E171 in 2008. A re-evaluation of the studies from 2015 to 2020 by the European Food Safety Agency (EFSA) in 2021 revealed a different picture . Due to the existing safety concerns, Titanium dioxide (E171) was banned as a food additive in the EU in August 2022.
There are scientific doubts about this ban. In 2022, the Canadian health authority concluded that there is no evidence of a carcinogenic effect. In Canada, titanium dioxide remains permitted in food (Health Canada, 2022). In the UK, there is also certainty that titanium dioxide has no such effect and the Food Standards Agency (FSA) https://www.food.gov.uk/ has no safety concerns and titanium dioxide also remains permitted there (COT, 2022).
Furthermore, international expert groups have doubts about the EFSA's evaluation and are calling for this decision to be reconsidered in the EU .
Uptake via medical applications
Titanium dioxide is contained in many medicines as a filler and excipient. Irrespective of the ban in foodstuffs, it may continue to be used in medicines as there is no alternative available and an immediate ban would lead to further bottlenecks in the supply and production of medicines.
However, the European Medicines Agency (EMA) has called on the pharmaceutical industry to look for alternatives as quickly as possible . The EMA is due to submit a report to the EU in April 2024 and the EU will then decide whether and how titanium dioxide may continue to be used in medicines (see also: EMA )
However, the quantities of titanium dioxide in the individual tablets or other dosage forms are very low. None of the many studies have so far been able to prove a recognisable risk from such low levels of titanium dioxide.
The intake of titanium dioxide via food is currently not given, but is still possible via medication. However, titanium dioxide is also absorbed from natural sources via food. To date, no valid study has been able to plausibly demonstrate a risk from orally ingested titanium dioxide. Titanium dioxide is not absorbed via the skin. Titanium dioxide can be absorbed via the lungs, primarily due to exposure in the workplace. However, the risk assessment of this material has been under discussion for several years (2018 to 2022). Titanium dioxide is regulated very differently around the world.
The effects of titanium dioxide nanoparticles have been studied in many plants and animals. They are thus among the most extensively tested nanoparticles. Both in vivo and in vitro data are available. Experiments have been carried out in various media (water, soil) and with different routes of exposure (water, food, blood, soil).
However, the studies are not easily comparable because the particle manufacturers and, thus, usually also the properties of titanium dioxide particles differ from study to study [1].
The rainbow trout as aquatic test organism has been very well studied and was confronted with titanium dioxide nanoparticles via the water, food, and the bloodstream. In coarser form (microscale), titanium dioxide particles have long been used in nutritional studies in fish and are considered nontoxic. TiO2 nanoparticles ingested via the food can be detected in the gills, intestine, liver, brain, and spleen. Thus, there is a systemic distribution of the particles in the body, but this had no effect on the health of the animals [2]. Titanium dioxide nanoparticles taken up directly from the water are only slightly absorbed into the fish's body [3].
In another study, rainbow trout were injected with TiO2 nanoparticles directly into the bloodstream and the distribution in the organs was observed. A non-environmentally relevant exposure, however, it serves to clarify mechanisms of action and effects on the uptake of very high doses, e.g. for a possible industrial accident [4]. The particles were enriched in the kidney and liver without affecting the functions of these important organs.
Zebrafish can also take up TiO2 particles from the water. The egg of fish embryos is not permeable for particles. If embryos are exposed to the particles in the presence of strong lighting, occurrence of malformations and increased mortality of the embryos is observed [5]. This effect does not occur under normal lighting conditions and is therefore due to the photocatalytic properties of the TiO2 particles. Adult zebrafish showed no effects after exposure to TiO2; the gills showed no morphological changes [6,7]. However, there were changes in the activity of certain genes; these changes were partly consistent with those observed after copper and silver nanoparticle exposure.
Water fleas (Daphnia magna) are among the most commonly used test organisms. In the often used two-day test, in which the daphnia are exposed for 48 h to the particles, no or only minimal effects (mobility, mortality) were observed in several studies [6,8,9,10]. However, when the observation period was extended to 3-21 days, effects on molting and reproductive ability became apparent, some leading to the death of all test organisms [8,9,10]. These indirect toxic effects are due, on the one hand, to the attachment of particles on the exoskeleton (carapace) of the animals, on the other hand, to particle absorption in the intestine. The latter may inhibit food intake in the chronic tests [10].
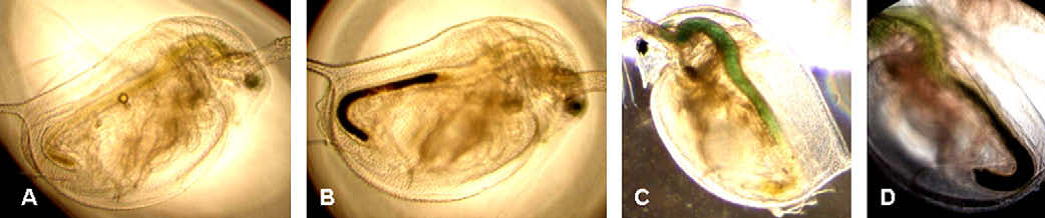
Water flea accumulate titanium dioxide nanoparticles (black coloured areas) in their gut. © Zhu et al., 2010.
An important question in risk research is the extent to which a transfer of nanoparticles through the food chain takes place. In a "small" food chain, consisting of water fleas and zebrafish, it was shown that transfer of nanoparticles from TiO2-fed daphnids to zebrafish occurs [11].
For other freshwater and saltwater organisms (mussels, snails) titanium dioxide nanoparticles were not acutely toxic [12,13], but the activities of certain enzymes showed a response to particle exposure [13,14].
Lugworms living in marine sediments did not internalize particles via the skin or the gut into the body tissue [15]. At very high concentrations, the worms’ food intake was reduced, a typical response to contaminants in the sediment. Also in high concentrations, TiO2 nanoparticles induced DNA and cell damage.
As an example of soil-dwelling organisms, woodlice were fed with titanium dioxide-soaked leaves. The nanoparticles had little influence on the metabolism and no effect on feed intake, body weight or mortality [16,17], although the concentrations used were very high. Similar to water fleas, however, longer duration of exposure had an influence on the effect of TiO2, an indication that unlike the commonly used short-term tests also chronic tests with longer exposure times should be performed. After 7 days of TiO2 exposure via the soil, a worm species showed DNA damage and evidence of oxidative stress, also in very high concentrations [18]. Similar observations were made for a nematode; here, also growth and number of offspring was reduced [19].
TiO2 nanoparticles were tested on various plants. In the onion and in willow trees, the toxicity was low and all growth parameters were unchanged [20,21]. Another study examined tobacco and onion plants; here, high, non-environmentally relevant concentrations caused genotoxic effects [22]. For a freshwater green alga exposed to 3 different TiO2 nanoparticles, growth inhibitory effects were observed, but these do not only depend on differences in particle sizes, but also on other characteristics such as different crystal structures [23]. Further, it is unclear, whether the nanoparticles hinder the necessary light and thereby inhibit algae growth.
In conclusion, from the studies available so far, a low toxicity of titanium dioxide nanoparticles to environmental organisms can be derived. Effects were always observed at concentrations well above the predicted environmental concentrations (PEC value).
The particles are taken up, however, without a doubt in organisms and cells, so we must consider for the future, that the effects of very low concentrations of these substances over a longer period, as it would comply with the conditions in the environment, have not yet been adequately examined (in Daphnia and woodlice).
Literature
- Menard, A et al. (2011), Environ Pollut, 159(3): 677-684.
- Ramsden, CS et al. (2009), Ecotoxicology, 18(7): 939-951.
- Federici, G et al. (2007), Aquat Toxicol, 84(4): 415-430.
- Scown, TM et al. (2009), Toxicol Sci, 109(2): 372-380.
- Bar-Ilan, O et al. (2012), Nanotoxicology, 6(6): 670-679.
- Griffitt, RJ et al. (2008), Environ Toxicol Chem, 27(9): 1972-1978.
- Griffitt, RJ et al. (2009), Toxicol Sci, 107(2): 404-415.
- Dabrunz, A et al. (2011), PLoS One, 6(5): e20112.
- Wiench, K et al. (2009), Chemosphere, 76(10): 1356-1365.
- Zhu, X et al. (2010), Chemosphere, 78(3): 209-215.
- Zhu, X et al. (2010), Chemosphere, 79(9): 928-933.
- Canesi, L et al. (2010), Aquat Toxicol, 100(2): 168-177.
- Musee, N et al. (2010), Chemosphere, 81(10): 1196-1203.
- Canesi, L et al. (2010), Aquat Toxicol, 96(2): 151-158.
- Galloway, T et al. (2010), Environ Pollut, 158(5): 1748-1755.
- Drobne, D et al. (2009), Environ Pollut, 157(4): 1157-1164.
- Jemec, A et al. (2008), Environ Toxicol Chem, 27(9): 1904-1914.
- Hu, CW et al. (2010), Soil Biol Biochem, 42(4): 586-591.
- Wang, H et al. (2009), Environ Pollut, 157(4): 1171-1177.
- Klancnik, K et al. (2011), Ecotoxicol Environ Saf, 74(1): 85-92.
- Seeger, EM et al. (2008), J Soils Sediments, 9(1): 46-53.
- Ghosh, M et al. (2010), Chemosphere, 81(10): 1253-1262.
- Hartmann, NB et al. (2010), Toxicology, 269(2-3): 190-197.
The effect of titanium dioxide nanoparticles has already been investigated in numerous plants and animals. This makes them one of the most extensively tested nanoparticles. In vivo and in vitro data are available and experiments have been carried out in various media (water, soil) and with various uptake routes (water, food, blood, soil).
Distribution and Effects in the Body
The effects in the lungs described above are acute reactions. There are still few studies on the chronic effects of nano-titanium dioxide particles on the body. Park and colleagues concluded from their investigations that titanium dioxide can possibly cause chronic inflammation of the lungs after instillation [. In principle, titanium dioxide particles act according to the „Granular biopersistent dust particles“.
Following the reassessment of titanium dioxide by the ECHA and EFSA , there were doubts as to whether titanium dioxide could have a tumor-inducing effect under certain circumstances and is therefore unsafe to use. In November 2022, the European Court of Justice classified the ECHA regulation as "wrongly adopted". After a detailed assessment of the published data, international expert groups also came to the conclusion that there is neither sufficient evidence of a harmful effect nor any indication of a possible carcinogenic effect of titanium dioxide zotpressInText item="{4274171:ENY9VPF7},{4274171:7ZNLS2HQ}"].
Behaviour at the blood-brain barrier
The brain is very well protected against the penetration of foreign substances by the blood-brain barrier. It is therefore unlikely that titanium dioxide particles will penetrate the brain. Particles could enter the brain directly via the olfactory nerve when inhaled. In a study on rats, it was shown that after injection of titanium dioxide nanoparticles into the bloodstream, there was no accumulation of the particles in the brain . Furthermore, no titanium was detected in the brain after titanium dioxide was injected into the lungs of mice .
Uptake and Effects in Cells
The majority of titanium dioxide particles are taken up into the cells by phagocytosis as large intracellular aggregates in vesicles, vacuoles or lamellar bodies (membrane-enclosed cell components). However, high doses disrupt phagocytosis, resulting in cell overload, e.g. in the lungs . Pinocytotic uptake was only observed for small aggregates (< 30 nm) and individual particles. After uptake, the titanium dioxide particles are usually membrane-bound in the cytoplasm of the cells. No particles have yet been found in the cell nucleus .
In vitro multi-cell systems (also known as co-culture systems) simulate the interaction of different cells in the body and thus the in vivo situation better than simple cell culture models with only one cell type. Using sensitive methods, it has been shown that titanium dioxide is present in all cell types of a triple co-culture system as membrane-bound larger aggregates, but also freely in the cytoplasm as smaller aggregates or individual particles .
In contrast, transcytosis, i.e., the transfer from one cell to another, has hardly been investigated in vitro. Measurements carried out as part of the NanoCare project on the transport of nanoparticles through cells showed that no transport of titanium dioxide particles takes place through single-layer cell layers . Exocytosis, a process in which substances are released from the cell into the cell environment, could not be observed in in vitro experiments.
Numerous in vitro studies with different cells show that cells react differently to exposure to titanium dioxide depending on their type and origin. Depending on the dose, titanium dioxide nanoparticles can induce the secretion of inflammatory markers, the formation of reactive oxygen species (ROS), cytotoxicity and apoptosis .
However, very high concentrations of nanoscale titanium dioxide (15 nm primary particle size) are necessary to irreversibly damage the cells .
In studies with human nasal mucosa cells and white blood cells no cell or DNA damage was detected, although isolated particles and increased agglomerates were present in the cells .
In the NanoCare project, titanium dioxide served as a so-called "reference material", i.e. it was used in all experiments. Studies with different cell lines showed that different variants of titanium dioxide only reduced the vitality of the cells after administration of very high doses (50 µg/cm2). This dose is not only far above the amount of naturally occurring titanium dioxide, but also above the dose resulting from the proper use of industrially produced titanium dioxide. In addition, titanium dioxide tends to clump strongly, which greatly reduces the number of free nanoparticles.
Using the so-called vector model, which depicts some of the elementary cell functions , it was shown that a concentration of approx. 60 µg particles per 106 phagocytes leads to damage to the cells. The formation of reactive oxygen species (ROS) was also only detected in the cells at this high dose zotpressInText item="{4274171:BA2AZD2Z}"].
As part of the NanoCare project, in vitro tests were also carried out using an exposure system developed at KIT for bioassays to determine the toxicity of gas-borne nanoparticles. Aerosol flows over the surface of cells and can cause a dose-dependent reaction in the cells, such as inflammation, due to the deposited particles. At the same time, the deposited particle dose per area is recorded using a quartz crystal microbalance .
Titanium dioxide was also used as a reference material in the BMBF-funded joint project INOS. The in vitro tests consistently showed that titanium dioxide had no cytotoxic effect on various human cell lines (A549, HaCaT, CaCo-2) and also on rainbow trout cells (concentrations of up to 50 µg/ml over 3 hours and 3 days).
In principle, most studies show no adverse health effects due to titanium dioxide. However, an increasing number of studies with very high concentrations have been carried out over the past 10 years. These and high-dose studies from the 1990s led the French Agency for Food Safety, Environment and Occupational Health and Safety (ANSES) to submit an application to the European Chemicals Agency (ECHA) to reclassify titanium dioxide. The ECHA followed this request and introduced new labelling for all products containing titanium dioxide. The European Food Safety Authority EFSA subsequently banned the use of titanium dioxide in food. The European Court of Justice overturned the labelling requirement due to a lack of evidence. Further decisions are expected in the future.
It is generally assumed that titanium dioxide (TiO2) particles regardless of their size do not dissolve. Thus it is likely that TiO2 retain their particulate form in the environment. Studies on the environmental behaviour of TiO2 nanoparticles were carried out under three main aspects: Influence of environmental conditions on the presence and mobility of the particles, binding of environmental contaminants by the particles, and the influence of particles on processes in the environment.
Studies on the influence of environmental conditions on the mobility are concerned in particular with naturally occurring substances in soil or water and their interaction with the nanoparticles. This is particularly the influence of agglomeration , as well as stability and deposition of particles. The natural materials include organic materials (degradation products of plants or animals), such as humic or fulvic acids, which are included in all waters and soils in different proportions.
In most cases, binding of such materials to TiO2 leads to stabilisation of the suspension particles and prevents their agglomeration [1,2,3,4,5,6,7]. As a result, particles rather "float" in the water, thus remain mobile and do not sediment. Stabilisation by organic materials is largely independent of the pH and salinity of the environment, i.e. takes place under various environmental conditions. The agglomeration-preventing effect of proteins is similar to that of organic materials [8].
However, certain substances like organic acids, e.g. oxalic acid, can also have opposite effects or may not exert any stabilising effect [9]. Minerals or salts also increase agglomeration of the particles [10] and reduce their mobility [1].
The behaviour of the particles is also influenced by their chemical and physical properties. Hence, contaminants (e.g. residues from production) may affect the surface charge and, thus, the behaviour of the particles [11,5]. However, according to the present studies, the different crystalline structures of TiO2 and different shapes and sizes have no effect on sedimentation and agglomeration of the particles.
In addition to the natural organic substances, also inorganic substancescan bind to titanium dioxide particles and thereby influence their behaviour in the environment and their effects on environmental organisms. Titanium dioxide particles can bind toxic heavy metals such as cadmium and arsenic [12,13,14].
Carp exposed to cadmium and arsenic-containing water and titanium dioxide particles took up more cadmium and arsenic than carp in particle-free water [13,14]. In algae, however, this effect was not observed, because algae do not absorb cadmium-laden particles [12]. Due to the photocatalytic effect of TiO2, arsenic is transformed into a less toxic form [15]. Similarly, an increased binding of phosphorus to TiO2 has been described [16].
Thus, TiO2 nanoparticles in principle bind many substances and increase the bioavailability for organisms. Whether TiO2 particles have a significant impact on the availability of other pollutants under field conditions has not been investigated yet.
TiO2 nanoparticles can also affect processes in the environment, e.g. by changing the properties of sediments with respect to surface area, pore size, and ability to bind to other substances [16]. This could for example increase the binding of nutrients in TiO2-contaminated soils. The precise effects of TiO2 enrichment, however, are still unexplored.
Also, water-purification processes in water treatment plants can be affected by high TiO2 concentrations. Effects on the removal of nitrogen compounds have been described [17]
Literature
- Ben-Moshe, T et al. (2010), Chemosphere, 81(3): 387-393.
- Domingos, RF et al. (2009), Environ Sci Technol, 43(5): 1282-1286.
- Keller, AA et al. (2010), Environ Sci Technol, 44(6): 1962-1967.
- Thio, BJ et al. (2011), J Hazard Mater, 189(1-2): 556-563.
- Von Der Kammer, F et al. (2010), Environ Pollut, 158(12): 3472-3481.
- Yang, K et al. (2009), Langmuir, 25(6): 3571-3576.
- Zhang, Y et al. (2009), Water Res, 43(17): 4249-4257.
- Allouni, ZE et al. (2009), Colloids Surf B Biointerfaces, 68(1): 83-87.
- Pettibone, JM et al. (2008), Langmuir, 24(13): 6659-6667.
- French, RA et al. (2009), Environ Sci Technol, 43(5): 1354-1359.
- Liu, X et al. (2011), J Colloid Interface Sci, 363(1): 84-91.
- Hartmann, NB et al. (2010), Toxicology, 269(2-3): 190-197.
- Sun, H et al. (2009), Environ Pollut, 157(4): 1165-1170.
- Zhang, X et al. (2007), Chemosphere, 67(1): 160-166.
- Pena, ME et al. (2005), Water Res, 39(11): 2327-2337.
- Luo, Z et al. (2011), J Hazard Mater, 192(3): 1364-1369.
- Zheng, X et al. (2011), Environ Sci Technol, 45(17): 7284-7290.