Zinc oxide is an all-rounder when it comes to technical applications: it is used in rubber production, in cement and paints, in electronics, in medical products and also as catalyst. Zinc is also an essential element indispensable to life of humans, animals and plants.
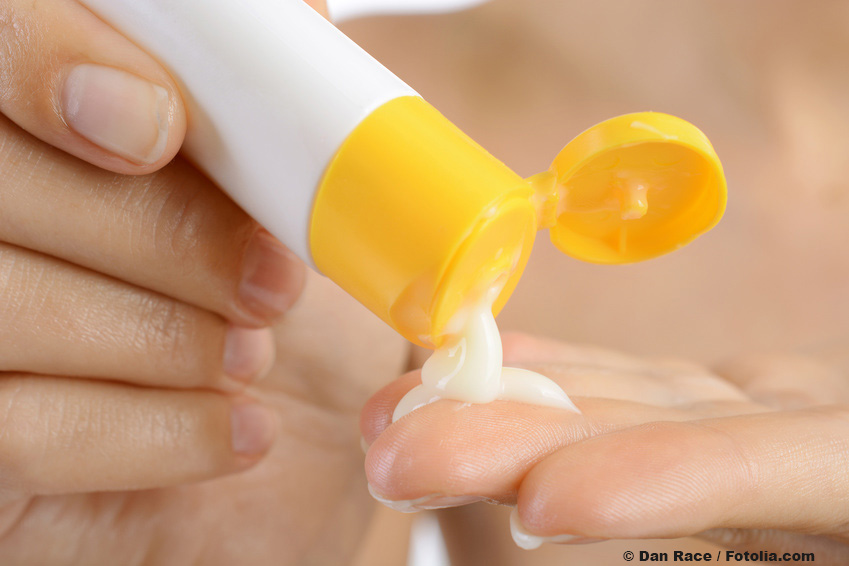
Skin lotion © Dan Race / Fotolia.com
How can I come into contact with this material?
For a healthy life, a human requires between 12 and 15 mg zinc on a daily basis obtained from the diet. In most cases zinc is taken up in form of zinc oxide coming from natural food sources which then dissolves in the body releasing zinc ions. But even zinc, if absorbed in too high concentrations, can be harmful to the body. A possible exposure scenario is the inhalation of zinc oxide fumes formed during welding activities of zinc sheets which can then lead to lung damage (metal fume fever). Zinc oxide nanoparticles are also used in cosmetic products as a mineral UV-filter. Dermal uptake of dissolved zinc ions can occur but has no potential harmful effect since the skin needs a lot of zinc for its own metabolism anyway. Most of our absorbed zinc comes from natural sources in the food.
Is there any risk from this material to humans and the environment?
Zinc as well as zinc oxide nanoparticles have a positive effect on the human body since zinc is involved in the regulation of many important biological processes. Therefore it is used in zinc ointments and other medical products. But if zinc is applied in high concentrations or in the wrong place (e.g. zinc oxide nanoparticles in the lung) it may have toxics effects causing cell death (zinc fever).
Conclusion
Humans are exposed to zinc oxide on a daily basis originating from various sources like food intake, cosmetics and many other products. Zinc is only harmful for humans in high concentrations as zinc is an essential element and zinc oxide dissolves once inside the body.
By the way…
- Zinc oxide is the 5th most common element in the earth’s crust.
- Zinc is also added to foodstuffs in the form of zinc acetate (E650) to prevent zinc deficiency in humans.
Properties and Usage
Zinc oxide (ZnO) forms colorless hexagonal crystals or a white dusty powder. When heated, its color turns into lemon yellow changing into white again when cooled down. Zinc oxide has a density of 5,61 g/cm³. It evaporates at 1300 °C and above and sublimates as it reaches a temperature of 1800 °C. Hence, there is no (liquid) melt but a direct transition from the solid into the gaseous aggregate state.
Zinc oxide has a very broad and versatile range of application including technical products, cosmetics, and pharmaceutical uses. At present, zinc oxide is selling strongest in the rubber industry. Used as additive, it promotes the process of vulcanization in rubber that is used for tire manufacturing. In addition, its good conductivity improves the removal of heat that is generated during the churning motion of the tires. When added to cement, zinc oxide increases the water resistivity and prolongs the processing time. Zinc dust and “zinc white” are also used as pore fillers in surfacers and smoothing cements and as gray or white wall or artist’s paints. Moreover, zinc oxide is used as catalyst in the chemical industry or for manufacturing cosmetic products. Zinc oxide together with ichors develops into antiseptic and astringent zinc salts, which is why it is used by the pharmaceutical industry for manufacturing zinc ointments, zinc pastes, adhesive tapes, and bandages for skin and wound treatment. There are different electronic components, for example piezo-electric converters, transparent conducting oxides, sensors, luminous diodes, and optoelectronic or spintronic components, that at present are barely conceivable without zinc oxide. Also, zinc oxide-based semiconductors are used as transparent conductive layers in blue light-emitting diodes, liquid-crystal screens, varistors, and thin-film solar cells .
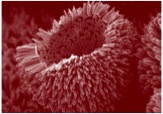
SEM image of nanoscale ZnO broken urchin © Empa
Nanoparticular zinc oxide is transparent in the visible range of the light spectrum and acts as a filter against the UV-B and UV-A radiation. The fact that the ultraviolet rays are absorbed and reflected like from small mirrors makes it interesting to use zinc oxide as a UV filter in sunscreens. Anorganic (mineral) filters are suited for application to the sensitive skin of children and allergic persons.
The sizes of zinc oxide particles used for sunscreens are in the range of 20 to 60 nm. Before being added, these very small zinc oxide nanoparticles are coated with silicon or aluminum oxide. They then coalesce into aggregates sized 200 – 500 nm. Studies conducted by the industry as well as independent studies carried out within the EU project NanoDerm have shown that such particles contained in sunscreen products do not penetrate the body through the healthy skin, hence are not hazardous to the health of consumers.
Since zinc oxide nanoparticles are characterised by their UV-A and UV-B protection properties as well as by the previously described transparency and antibacterial and fungistatic effects, they are also used in textiles, clear varnishes for wood products and furniture, and in transparent plastics and plastic films (plastic glasses). The latter are characterised by a high transparency (> 90 % transmission) in the visible spectral range and UV permeability (< 10 % transmission) for wavelengths below 360 nm.
Zinc oxides are not self-inflammable as nanometer-sized powders. The mixture of zinc oxides and air (dust) under the influence of an ignition source is not flammable, so there is no possibility of dust explosion.
Occurence and Production
Zinc oxide (ZnO) occurs in nature as a coarse-grained mineral (zincite). Technically, it is obtained by oxidation of zinc or zinc vapor with atmospheric oxygen (zinc white) or by calcination of different components such as zinc hydroxide, zinc carbonate or zinc nitrate. Technical zinc oxides often contain lead. Such plumbiferous compounds must be marked with the corresponding hazard symbol (Xn).
NanoCare Data Sheet
- Zinc Oxide data sheet No.1 (PDF )
Additional Information
- Roempp-online.de (DE): Zinkoxid (last access date: Jul 2011).
- EU-Project NanoDerm (last access date: Jul 2017).
- BASF Infoservice (EN): The new generation of UV filters: reliable all-round protection for the whole day.
- BfR Opinion No. 037/2010 (18.06.2010). Sunscreen: According to the current stat e of knowledge zinc oxide as UV filter is safe (PDF ).
Zinc oxide is found in sunscreen, textiles, paints or even plastics. In high doses, it can have a toxic effect.
General hazard
It is a fact, however, that inhalation of zinc oxide fumes during welding may cause metal fume fever . Bearing the symptoms of influenza, this disease gets better after a few days but may impede lung function if exposure is continued. For all that, it is much more probable that, being used as anorganic (mineral) UV filters in suncreams and in cosmetics, the zinc oxide nanoparticles are taken up via the skin.
Studies on Living Organisms – in vivo
Administration of fine or nanoscale zinc oxide particles into the lungs of mice or rats was found to cause severe but temporary pneumonia . Quite interestingly, both the intensity and course of this reaction were practically identical for fine and nanoscale zinc oxide.
Studies Outside the Body – in vitro
Most of the in vitro studies carried out reveal a relatively high toxicity of zinc oxide nanoparticles for cells of different tissues and different organisms. The very steep dose-effect curves found for the ZnO particles indicate that from a certain concentration, the toxic effect increases very rapidly. For most cell types, the relevant value is in the range of 10-20 µg/ml.
The studies carried out within the project NanoCare on eleven different cell lines of different origins show that these cell lines are differently sensitive to the ZnO particles. Here, too, a relatively high toxicity was found already at low concentrations in some cell lines (LOEL from 5g/cm2 or approximately 16µg/ml). For some cells, the threshold values for in vitro apoptosis tests were in the range of 7,5-10µg/cm2 .
In addition to simple culture systems with only one cell line, also complex so-called co-culture systems were used within NanoCare. Using such systems, the in vivo situation in the body can be displayed better due to simulation of the interaction of the cells. In these systems, zinc oxide particles were found to cause increased levels of inflammation markers .
The mechanism responsible for the high toxicity of zinc oxide particles has not yet been completely clarified. It seems, however, that released zinc ions and reactive oxygen radicals are playing an important role . Likewise, it is not yet clear whether the observed toxic effect is influenced by the shape and size of the zinc oxide particles. According to several studies, however, there are no traceable size-dependent effects .
Coating with gold or aluminum was found to strongly reduce the toxicity of the zinc oxide nanoparticles . It was postulated, therefore, that the cellular effects are rather caused by electronic properties or solubility than by the size of particles. zinc oxide nanoparticles are of interest to medical applications due to the possibility of changing their toxicity through selective modifications . Since zinc oxide nanoparticles seem to be very toxic to cancer cells, (that is, also to many cell lines used in cell culture systems) but not to normal cells , they are investigated, in addition, for their potential cancer fighting capability and their ability to serve as drug-administrating vehicles.
The use of nanoscale zinc oxide (ZnO) in cosmetics, coatings and cleaning agents is expected to result in a relatively high load in surface waters compared to other nanoparticles. Zinc oxide particles dissolve very rapidly in liquids. Actually measured environmental concentrations are not yet available for zinc oxide nanoparticles.
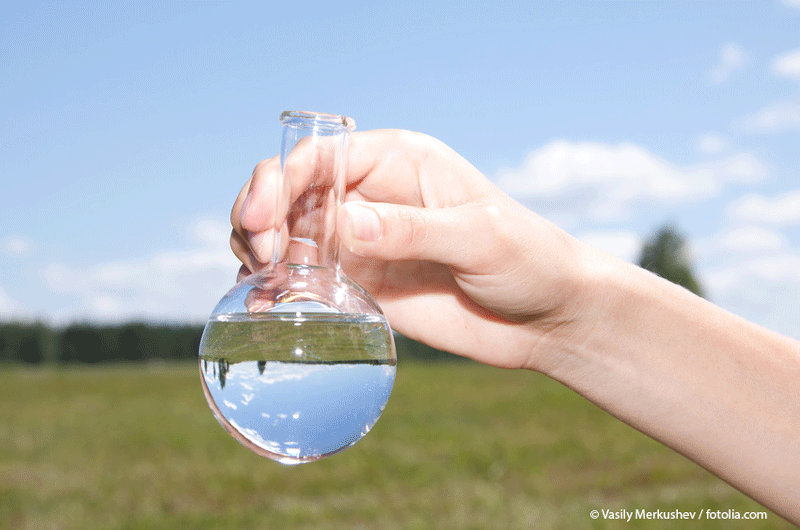
Water sample © Vasily-Merushev/Fotolia.com
The expected zinc oxide nanoparticle concentrations in the environment were estimated using computer models. Compared to other nanomaterials (e.g. gold), the expected zinc oxide concentrations are very high. This results from many applications, such as textiles or sun screen, from which the zinc is released directly into the environment after use. Only a small share of zinc oxide nanoparticles released into the environment reaches the air. The concentrations of zinc oxide nanoparticles in European waters are subject to severe fluctuations, depending on population and sewage plant density, as well as the seasons. Less zinc oxide is expected to occur in soil and air .
Depending on the environmental compartment, and in relation to known toxic concentrations for aquatic organisms, risk quotients of less than 1 up to ~10 are calculated. This is an indication that an environmental risk by zinc oxide nanoparticles cannot be ruled out for certain compartments (e.g. soil after application of sewage slugde). However, these theoretically calculated values need confirmation in the future by experimentally collected measured values .
Due to its applications and its high solubility, zinc oxide nanoparticles are expected to occur mainly in surface waters, whereas less zinc reaches air and soil.
During the production and processing of zinc oxide nanoparticles, there is a possibility of ingestion by inhalation. No particles from sunscreen products enter the body through healthy skin, but could be absorbed by ingestion.
Uptake via the Lung - Inhalation
First animal model studies show that inhalation of Zinc oxide nanoparticles may effect a relatively strong but temporary inflammation of the lung . This resembles the so-called metal fume fever, an influenza-type disease characterized by inflammation of the respiratory tract due to inhalation of metal fumes (mainly zinc fumes) during welding.
Uptake via the Skin – Dermal Uptake
Since zinc oxide nanoparticles are used as UV protection in suncreams and cosmetics, it is very important to know whether they can be taken up via the skin. The size of the zinc oxide particles used in suncreams is in the range of 20 to 60 nm. Before being added, these very small nanoparticles are coated with silicon or aluminum oxide to clog up to form aggregates sized 200 to 500 nm.
Studies from industry and independent studies carried out within the EU project NanoDerm have shown that such particles do not get into the body through the healthy skin. Hence, the users of such sun protection products do not incur health risks .
Most of the studies carried out so far, however, were based on cell cultures or animal models. Some recent tests of ZnO-containing suncreams on humans under realistic conditions, indeed, revealed small quantities of marked zinc in the blood and urine . The quantities detected only amounted to 1/1000 of the zinc concentration naturally occurring in the blood. It remains to be found out whether zinc was taken up via the skin as zinc oxide particles or dissolved zinc ions.
Uptake via the Gastro-Intestinal Tract
In a study of mice, increased concentrations of zinc were found in the liver, heart, spleen, stomach, and the bones after oral administration of zinc oxide nanoparticles .
The element zinc is essential for all living things in small doses, but is known to be toxic in high doses. This observation is reflected in the results on the toxicity of zinc oxide nanoparticles. In general, zinc oxide particles dissolve very rapidly in liquids, so that zinc ions are always present in addition to the particles. For zinc oxide comparative investigations have been carried out in various test organisms on the action of zinc salts, micro- and nanoscale particles.
Zinc and zinc oxide are known to be antibacterial effective (e.g. in zinc ointments) and consequently zinc oxide nanoparticles inhibit the growth of various bacterial species. It is assumed that the toxicity largely depends on the released zinc ions. Bacterial growth inhibition was enhanced under the influence of light, and was more pronounced for smaller particles compared to larger ones. Oxidative stress and damage to the outer membrane is thought to be a mechanism of toxicity. In addition, zinc oxide nanoparticles damage the genetic material in some types of bacteria. Due to the different sensitivity of individual bacterial species, bacterial communities change in their composition under the influence of zinc oxide nanoparticles. The harmful effect of high doses of zinc can affect the many important functions that bacteria take in the environment, such as the nitrogen and methane fixation .
The general signs of damage of fish due to excessively high zinc concentrations range from developmental delays to the damage of individual organs or the immune system. More detailed studies of the mechanism of action revealed the formation of oxidative stress by zinc oxide particles as a possible cause of toxicity. Some fish species, but not all, internalize zinc oxide nanoparticles and zinc was detected in various tissues (liver, brain, gills). In numerous fish studies, zinc oxide nanoparticles produced stronger effects than zinc ions. In some studies, no difference was found. In any case, a part of the effect is attributed to the dissolved ions, which can obviously be amplified by the particles .
Zinc exerts toxic effects in water fleas. Organic substances that occur naturally in water can reduce the toxic effect of the zinc oxide nanoparticles. Since water fleas filter the water for food intake, nanoparticles enter directly into their bodies, but an efficient excretion from the gut was observed. In water fleas, zinc oxide nanoparticles exerted the same toxic effects as zinc ions. It was also described that the more ions particles release, the more harmful they were.
Similar observations for zinc oxide nanoparticles have also been made in a number of other crustaceans and zooplankton. The effect of zinc oxide nanoparticles and zinc ions did not differ in isopods when the effects were observed at the whole-organisms level .
The influence of zinc oxide nanoparticles on the growth, reproduction and behaviour of threadworms was comparable to that of zinc ions, however some results also suggest a stronger effect of smaller particles. The particles also cause oxidative stress in threadworms. Light can increase the toxicity of zinc oxide nanoparticles. In earthworms, zinc oxide nanoparticles produce similar effects as in threadworms. However, in this organism, ions have a greater toxicity than nanoparticles .
Zinc oxide nanoparticles are absorbed by mussels and snails and are toxic in high concentrations. Here too, oxidative stress has been demonstrated as a mode of action. Zinc is also toxic to sea urchin embryos. Here the released ions are primarily responsible for the toxicity of the particles. Another study shows that the nanoparticles are more toxic than the ions. In addition, it has been shown that the nanoparticles alter the eggshell, which could facilitate uptake of other contaminants into the embryo.
Claw frog embryos also internalise zinc oxide nanoparticles. The particles trigger oxidative stress, resulting in damage to cells .
Zinc oxide nanoparticles influence the nervous system and lower the survival rate of bees. In jump tails, zinc oxide nanoparticles and zinc ions likewise cause damage in the genome and reproductive capacity. The size of the particles has no influence on the toxic effect. The animals avoid soil contaminated with zinc .
For algae, zinc is a toxic element, the active component being the zinc ion. Both zinc oxide nanoparticles and coarser particles liberate these ions in different proportions, and are therefore toxic to algae. The extent to which harmful effects depend on the shape and size of particles is evaluated differently. Some studies do not find any dependence, while others observe a dependence on both the shape and the size of the particles. Zinc causes a reduced growth rate and oxidative stress in algae. Zinc oxide nanoparticles are absorbed onto algal cells .
Zinc can also be toxic to plants, whereby the individual species show different sensitivity. The ions liberated from the nanoparticles are mainly responsible for plant toxicity. Besides a general growth retardation, the roots of the plants are impaired because they have the first contact with the zinc oxide nanoparticles. A delayed germination was observed for seeds. Zinc is absorbed into plants and is distributed more or less into roots, shoots, leaves and fruits. Zinc oxide nanoparticles also cause the formation of oxidative stress in plants .
Many studies, but not all, attribute the detrimental effects of zinc to the release of ions from the nanoparticles. Overall, particles of different sizes and zinc ions elicit similar symptoms, for example oxidative stress, which has been detected in almost all the organisms studied. The overall toxic potency differs depending on the organisms’ sensitivity.
Additional Literature
- Further evaluated literature for the nanomaterial zinc oxide can be found here (PDF, 85 KB )
In the aqueous milieu of the human organism, zinc oxide nanoparticles partially or completely dissolve and zinc ions are released.
Behaviour of uptake in somatic cells
The process of dissolution, however, is not equally efficient for the different particles and depends, moreover, on the exact composition of the aqueous solution. Hence, the stability of the nanoparticles in the respective environment seems to decide on the uptake by the cells of either zinc oxide particles or dissolved zinc ions. In the literature, one therefore finds both studies describing the uptake of zinc oxide particles in the cell and studies where particles are not reported . Uptake of particulate zinc oxide followed by dissolution in the cell is expected to cause very high local concentrations of zinc ions accompanied by nanoparticle-specific reactions.
Zinc oxide nanoparticles are positively charged and belong to the group of soluble nanoparticles. They show a strong agglomeration tendency and are prone to dissolving meaning that in addition to the actual nanoparticles there are always dissolved zinc ions present in aqueous solution. Most data on zinc oxide nanoparticles is available for their behaviour in water and soil, whereas up-to-now no information could be retrieved for air.
In normal tap water, zinc oxide nanoparticles are not very stable and sedimentation occurs. When adding humic acid, a substance occurring in natural waters, the particles are stabilised and their mobility increases. The solubility of the nanoparticles depends on particle properties such as size, but also on the nature and composition of the surrounding environment. Under the conditions prevailing in a waste water treatment plant, zinc oxide nanoparticles are assumed to completely dissolve. Thus, factors such as ambient temperature and pH influence strongly agglomeration and ion release. The nature of the surface coatings can also influence the solubility of zinc oxide nanoparticles .
Zinc oxide nanoparticles entering sediments or soils are considered to be poorly mobile, which means they remain more or less at the site of their entry into the environment. Phosphates, such as those found in groundwater, however facilitate transport of zinc oxide nanoparticles .
Zinc oxide nanoparticles are photocatalytically active and can be used to degrade chemical substances in the environment .
Zinc oxide nanoparticles are considered less stable under environmental conditions since they have a strong tendency to dissolve. The speed of the process is dependent on the prevailing environmental conditions. Due to their tendency for sedimentation, a large share of zinc oxide nanoparticles released into the environment deposits in sediments.