As a car exhaust catalyst, cerium dioxide in the form of nanoparticles can convert highly toxic carbon monoxide into less harmful carbon dioxide. The semiconductor industry uses nanoparticles of cerium dioxide in the manufacture of computer chips: Here, they serve as an ultra-fine grinding and polishing agent, comparable to the tiny grains on fine sandpaper.
Non-nanoscaled cerium dioxide can improve the light production/output in mantles of gas lanterns as the gas flame produces almost no light itself, whereas burning cerium oxide generates a yellowish-white colour.
How can I come into contact with this material?
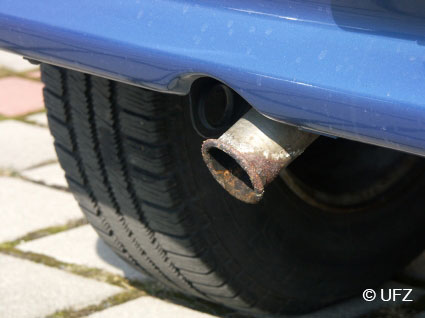
car exhaust pipe © UFZ
Ceria nanoparticles may be present in the ambient air as the material is used as a catalyst/additive in some automotive fuels. However, there is no information available on this material with regards to the environment or on other sources that may release cerium dioxide nanoparticles. The chance of human exposure to ceria nanoparticles derived from applications such as gas lanterns or computer chips is very low since mantles in gas lanterns are rarely produced nowadays and they do not make use of cerium dioxide nanoparticles at all. Equally the production of computer chips during which ceria is uses as polishing agent takes place in highly isolated rooms.
Is there any risk from this material to humans and the environment?
Little information exists on the effects of cerium dioxide nanoparticles on humans or the environment. Literature sources show that there could be positive and negative effects. There is no danger associated with small amounts of cerium dioxide. Currently it is also assumed that very little nano-scaled cerium dioxide exists in the environment.
Conclusion
Future studies need to be performed to conclusively determine the effects of cerium dioxide nanoparticles on humans and on the environment. Currently various international research projects are focused on investigating the effects, including the long-term effects, of cerium dioxide nanoparticles.
By the way…
Cerium, as cerium oxide, is a rare metal and was named after the dwarf planet Ceres that is located in the asteroid belt between Mars and Jupiter. It is not clear if either cerium or cerium dioxide is present on Ceres. The NASA probe Dawn, who arrived at Ceres in February 2015, could not clarify this: cerium was simply not on the agenda for this research program.
Properties and Applications
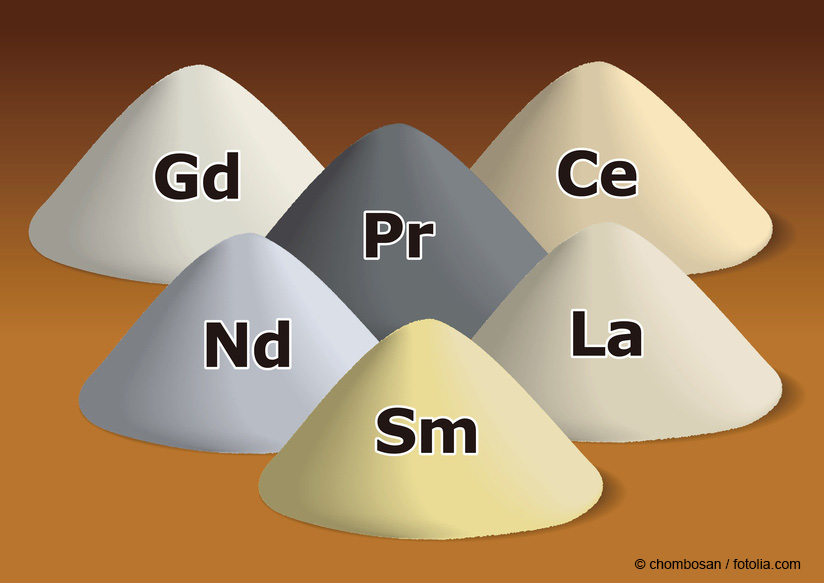
Rare-earth elements © chombosan / fotolia.com
Cerium dioxide (CeO2) is an oxide of the rare-earth metal Cerium (chem. Ce), which belongs to the lanthanide group. Technical grade CeO2 is generally present as micro- or nanoscale white to light yellow powder [1].
In applications cerium dioxide gives infrared filters their anti-reflection coatings and is found in colour television tubes. Together with cobalt it serves the production of powerful magnets. As a consequence of that cerium glows intensely as soon as it is warmed-up, it is also used for incandescent mantles, combined with other rare-earth metal oxides. This leads to a considerably improved light yield of gas lamps.
In addition, cerium dioxide is used as a polishing agent for optical glasses and is applied as a grinding medium for computer parts, camera phone lenses, or CD player lasers.
A huge market for nano-structured CeO2 is cleaning and polishing of silicon wafers, which are required by the electronical industry for ultra modern chip systems and solar cells. Due to the reaction of CeO2 with the processed material, it can be removed in a way extremely fine dosed which enables the manufacturing of ultra smooth surfaces, which pose the basic prerequisite for miniaturizing circuits. Together with aluminium oxide, cerium dioxide constitutes the material of first choice in the matter of chemical-mechanical polishing (CMP) [2].
Nano-structured cerium dioxide, among other things, is used as an oxygen-storing diesel additive in vehicle exhaust catalysts. It still oxidises carbon monoxide and excess hydrocarbons into CO2 when there is a temporary lack of oxygen in the exhaust mixture. During that process, CeO2 is reduced to Ce2O3 that is re-oxidized later as soon as there is enough oxygen again in the exhaust gases. Aside from its role in exhaust catalysts where it mainly supports the much more active but also more expensive platinum, there is also a direct addition to the fuel intended. The cerium dioxide particles are supposed to reduce the fuel consumption as well as the emission of soot particles of diesel engines [3,4,5].
In fuel cells with other rare-earth metal oxides coated CeO2 particles are applied to improve the oxygen ion conductivity and so to enable reduced operating temperatures.
Moreover, nano-structured CeO2 poses an ideal UV absorber and is therefore used as an additive in lacquers and coatings for wood preservatives to enhance their UV stability.
Cerium dioxide is not self-inflammable as nanometer-sized powder. Also as a mixture with air (dust) under the influence of an ignition source, cerium dioxide is not inflammable, so there is no possibility of a dust explosion.
Natural Occurrence and Production
Cerium, named after the planet Ceres, can relatively often be found in nature in minerals like cerite, monazite or bastnaesite (ranked 28th in elemental abundance). In combination with oxygen it is easily converted into CeO2. Apart from CeO2 cerium forms the oxides Ce2O3 and C3O4[1]. Normally cerium dioxide is the result of the oxidation of cerium hydroxide and cerium carbonate.
NanoCare - Data Sheets
- Cerium Oxide data sheet No.1 (PDF)
- Cerium Oxide data sheet No.2 (PDF)
- Cerium Oxide data sheet No.3 (PDF)
- Cerium Oxide data sheet No.4 (PDF)
- Cerium Oxide data sheet No.5 (PDF)
Literature
- Römpps Chemie-Lexikon (DE), Band 1, 8. Aufl. 1979, Franckh’sche Verlagshandlung, W. Keller & Co, ISBN 3-440-04511-0.
- Gutsch, A et al. (2005), Chemie Ingenieur Technik, 77(9): 1377-1392.
- Technology Review (2006). Cleaning up combustion? Peter Fairley, 28.08.06. (last access date: Feb 2019)
- Sajith, V et al. (2010), Adv Mech Eng, 2010 1-6.
- Selvan, V et al. (2009), ARPN Journal of Engineering & Applied Sciences, 4(7) (PDF ).
Cerium dioxide is used in various applications, e.g. in fuel cells, wood preservatives, paints or abrasives and polishes.
General Hazard
Cerium dioxide is used in various applications, e.g. fuel cells, wood preservatives, varnish or abrasives and polishing agents. There are actually no studies on general hazard, thus no epidemiological data are available.
Literature
- Li, Y et al. (2016), Environ Toxicol Pharmacol, 44: 25-29.
Studies on Living Organisms – in vivo
Within the project NanoCare short-term inhalation studies (6 hours per day, over 5 days) have been carried out with rats to investigate the adverse effects of cerium dioxide nanoparticles. In this study the inhaled particles could be found within the lung as well as in the lung-associated lymph nodes. Neither in the liver, the kidneys, the spleen nor within the brain and the olfactory bulb any particles could be found [1]. Further investigation of the lung lining fluid could demonstrate the appearance of inflammatory markers after the exposure to CeO2 nanoparticles dependent on the dose administered.
After exposure of animals to cerium dioxide via the food or directly into the blood stream, the particles can be found in various organs such as the liver or the heart. The scavenging effect of oxygen radicals leads to a protective effect of CeO2 for nerve cells [2].
Literature
- NanoCare 2009, Final Scientific Report, ISBN 978-3-89746-108-6. (PDF-Document, 19 MB ).
- Hirst, S.M. et. al. (2013), Environ Toxicol, 28(2): 107-118.
Studies Outside of Organisms – in vitro
Various in vitro studies with nanoscale cerium dioxide particles could demonstrate that these metal oxide nanoparticles induce different responses within the cells dependent on the cell type investigated [1, 2]. For human lung epithelial cells (BEAS-2B) treated in vitro with various metal oxide nanoparticles it has been shown that these pure metal oxides could not induce inflammatory mediators [3]. On the contrary, in the human lung epithelial cell line A549 the cell viability decreased in one study dependent on the particle concentration [4], whereas another study could not observe such a cytotoxic effect by nanoscale cerium dioxide [5]. The human immune cell line Jurkat could be protected by CeO2 against cell-damaging UV-light [6]. The same protection effect could be demonstrated for various lung cells of rats. In this case the cerium dioxide nanoparticles serve as antioxidant and reduce the production of reactive oxygen species (ROS) [7].
Only a small number of studies found toxic effects induced by CeO2 in cells in culture [1, 4]. Most common is the induction of oxidative stress responses, which may be followed by cell death. On the other hand, studies demonstrating exactly the opposite, are in majority. Because of its chemical properties it can be observed that CeO2 prevents the production of oxygen radicals and protects by this the cells from cell death [6-8]. Within a series of in vitro experiments with the human lung epithelial cell line A549 the project consortium NanoCare has described a relatively high threshold limit for toxicity of CeO2. Only such high concentrations (defined as lowest observed effect level - LOEL) can induce stress responses as well as the production of inflammatory marker and reactive oxygen species (ROS). Lower concentrations of cerium dioxide could not induce any toxic effect in different cell lines [9]. This was confirmed by a recent review, which presents an overview about the important studies on various nanomaterials [2]. Herein it is mentioned that the size of the CeO2 particles is not important for an effect. Moreover, the toxicity is described as very low, which is possibly a result of the protective effect against ROS.
Additionally to the very simple monoculture system with only one cell line the project NanoCare used also more complex co-culture systems [9]. Such systems compare much better to the original situation within the organism as the interaction between different cell types can be simulated. It could be demonstrated that co-cultures respond more sensitive to CeO2 compared to monoculture. This may be the result of communication between the cells which can enhance the overall reaction.
Literature
- Mittal, S. & Pandey, A.K.(2014), Biomed Res Int, 2014891934.
- Simko, M. et. al. (2015), Int J Mol Sci, 16(11): 25954.
- Veranth, J.M. et. al. (2007), Part Fibre Toxicol, 42.
- Lin, W. et. al. (2006), Int J Toxicol, 25(6): 451-457.
- Park, B et al. (2008), Inhal Toxicol, 20(6): 547-566.
- Caputo, F. et. al. (2015), Nanoscale, 7(38): 15643-15656.
- Dunnick, K.M. et. al. (2015), Biol Trace Elem Res, 166(1): 96-107.
- Hirst, S.M. et. al. (2013), Environ Toxicol, 28(2): 107-118.
- Nanocare (2009). Report:"Final Scientific Report", Dechema e.V., Frankfurt a.Main. ISBN:978-3-89746-108-6 (PDF, 19 MB ).
Real occurring concentrations of cerium dioxide nanoparticles (CeO2) in the environment are not yet known. But as an alternative, the expected environmental cerium dioxide concentrations (PEC value) can be predicted using specific computer models.
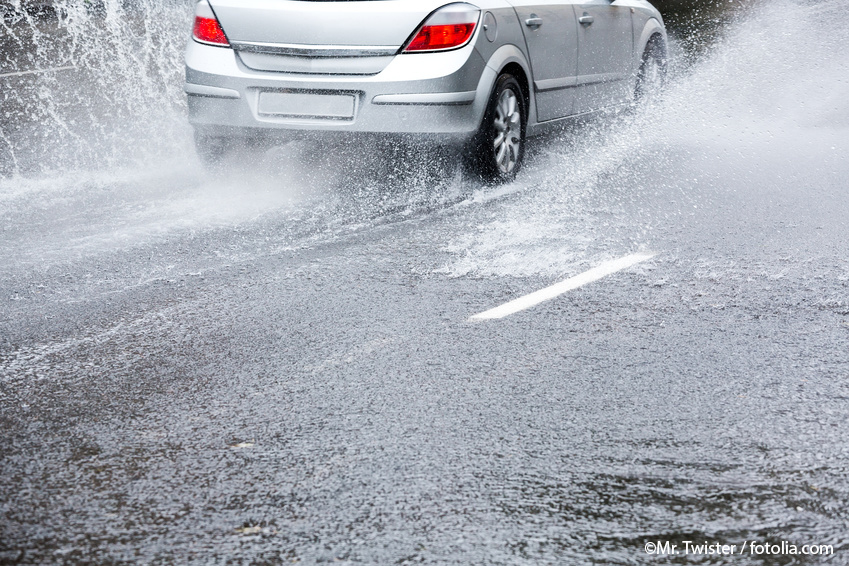
Road run-off due to rain event © Mr. Twister / fotolia.com
The estimated release of cerium dioxide nanoparticles is in the range of 1000 - 1500 metric tons/year, which is significantly lower than other nanomaterials like titanium dioxide (38000 metric tons/year). Ceria nanoparticles are often applied in closed systems, e.g. for polishing wafers, where no release takes place.
The majority of released cerium dioxide nanoparticles is anticipated to occur in soil and landfills. By some estimation, approximately 0.0001 µg/l and 0.01 µg/kg from consumer products or diesel exhaust containing ceria nanoparticles will reach surface waters and soil, respectively. The values of ceria nanoparticles in soils located besides major roads in Great Britain were predicted to be 0.016 µg/kg. Due to draining water along the road surface, the water may contain up to 0.3 µg/l of cerium dioxide nanoparticles [1-4].
Overall, compared with other nanomaterials (e.g. TiO2 , Fullerenes, ZnO ) and many other chemicals, the expected environmental concentrations (PEC value) of ceria nanoparticles are very low
Literature
- Keller, AA et al. (2013), J Nanopart Res, 15(6).
- Tiede, K et al. (2009), J Chromatogr A, 1216(3): 503-509.
- Boxall ABA et al. (2008), Report: "Targeted monitoring for human pharmaceuticals in vulnerable source and final waters", Drinking Water Inspectorate Project No. WD0805 (Ref DWI 70/2/231)
- Johnson, AC et al. (2012), Environ Toxicol Chem, 31(11): 2582-2587.
Studies show that cerium dioxide nanoparticles can enter the body. On the one hand, they can trigger inflammatory processes, on the other hand, they can have a protective effect on the body.
Uptake via the Lung – Inhalation
After inhalation of particles these can be determined within the lung but also in other organs of the exposed animals but only in very small amount (read also body barriers - Nanoparticles and the lung). The major part of the nanoparticles stays within the lung, only less than one per thousand of the applied dose could be found after 28 days within the liver, the spleen and the kidneys of the treated animals. These small amounts do not have any adverse effect within these organs. These studies demonstrated further that the size of the investigated particles doesn’t have an influence on the uptake and distribution of the particles within the organisms [1-3].
Additionally, the shape of the nanoscale cerium dioxide influences the biological effects. A study on the comparison of spherical and rod-like CeO2 nanoparticles in mice showed that only the rod-like particles with a minimum length of 1 µm could induce a sustained inflammation in the lung. Although the shorter rods (length < 1 µm) as well as the spherical particles could induce inflammatory responses, these are transient and disappeared after a short period of time [4]. The researcher of this study comment on their own study like this: ”When comparing the results of this study with those carried out with other metal oxide nanoparticles, cerium oxide has to be classified as low toxic. ....”
Animal studies on inhalation of cerium dioxide nanoparticles demonstrated the lung burden and the induction of inflammation in dependency on the concentrations and the shape of the particles. Results on possible long-term effects of CeO2 nanoparticles are expected at the end of 2018. At that time a subchronic inhalation study is finished.
Literature
- NanoCare (2009). Report:"Final Scientific Report", Dechema e.V., Frankfurt a.Main. ISBN:978-3-89746-108-6. (PDF-Document, 19 MB ).
- Geraets, L. et. al. (2012), Toxicol Sci, 127(2): 463-473.
- He, X et al. (2010), Nanotechnology, 21: 285103
- Lin,S. et. al. (2014), ACS Nano, 8(5): 4450-4464
Uptake via the Skin - Dermal Uptake
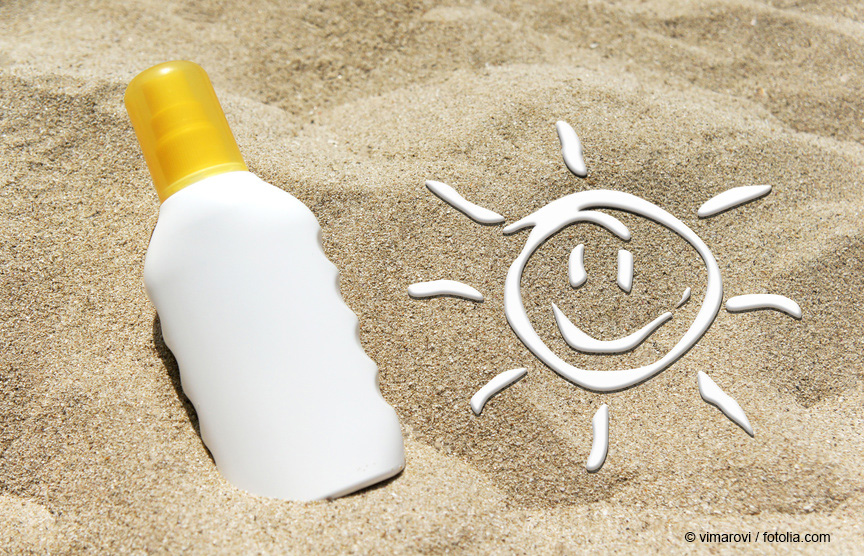
©vimarovi / fotolia.com
The uptake of cerium dioxide particles via the skin is related mainly to cosmetics or pharmaceutical products, e.g. sunscreens. Because CeO2 possesses UV-filter properties but also antioxidative activities it is under investigation as a new inorganic (also called mineral) UV-blocking agent in sun protecting creams. So far there are no real products on the market available [1].
Literature
- Caputo, F. et. al. (2015), Nanoscale, 7(38): 15643-15656.
Uptake via the Gastro-Intestinal Tract
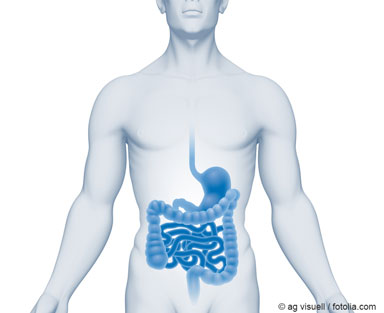
© ag visuell / Fotolia.com
In recent years various experiments on the uptake of cerium dioxide by the gastrointestinal tract (GIT) have been carried out. The aim of these studies was to investigate possible damaging effects of these particles after the uptake into the body. Most of the studies did not observe any adverse effect after exposure via the GIT. Moreover, very sensitive analyses have been done with radioactively labelled cerium dioxide which can be detected within the body in very small amounts. By using this technique, it could be demonstrated that very small particles with a diameter of around 7 nm could not be translocated through the epithelium of the intestine. More than 90% of the applied dose left the body via the faeces within one day, the remaining amount followed within two further days which results in nearly 100% excretion after three days. After overdosing experiments only a very small amount of 10 billionth could be found in the blood stream [1, 2].
After injection of cerium dioxide nanoparticles directly into the blood stream the particles could be found in the liver and the spleen, whereas no particles have been observed in the brain and the heart. The injected CeO2 has diminished the damaging effect of a liver toxin without inducing an adverse effect by its own. The same result has been shown for application via the food.
Another study investigated the exposure of fishes with different shapes of cerium oxide nanoparticles [3]. The result was very interesting as the spherical nanoparticles did not induce any toxic effect whereas the longest rods could induce inflammatory responses in the animals.
An animal study on rats could demonstrate a negative response of the animals [4]. Relatively high concentrations applied with the food in an acute-toxic study design induced slight DNA-damages but only at the highest dose of 1000 mg/kg body weight and this response was transient as after 48 h the damage decreased. In another comparable repeated-dose study for 28 days [5] the effects have been much stronger but as shown before only at the highest concentrations.
Literature
- He, X. et. al. (2010), Nanotechnology, 21(28): 285103
- Hirst, S.M. et. al. (2013), Environ Toxicol, 28(2): 107-118
- Lin, S . et. al. (2014), ACS Nano, 8(5): 4450-4464
- Kumari, M. et. al. (2014a), Mutat Res, 775-7767-19
- Kumari, M. et. al. (2014b), Mutagenesis, 29(6): 467-479
In previous studies, cerium dioxide nanoparticles showed toxic effects towards environmental organisms. Size-dependent toxicity of cerium dioxide nanoparticles, meaning the smaller particles exhibited the stronger effects, was only found occasionally.
Microorganisms show different reactions towards cerium dioxide nanoparticles. Whereas ceria nanoparticles (CeO2) are not toxic for yeast or bacteria from sewage sludge, they cause toxic reactions or growth inhibition in various bacteria e.g. occurring in soil or those associated with plants or biogas production. However, the mechanism of particle-mediated toxicity is not yet fully understood [7,8,11-19].
Cerium dioxide nanoparticles disturb the growth and metabolic activity of algae size-dependently. This is either due to the cell wall being damaged by the nanoparticles or by impairing nutrient uptake. Polymer surface coating of ceria nanoparticles can minimise the nanoparticles' toxic effects and also the binding to natural organic material lowers the toxicity by reducing the particle binding on the cell surface [3,11,17,19-27].
Water fleas swimming in water containing cerium dioxide nanoparticles show no adverse effects with respect to mortality or mobility but there exist species-specific variations in sensitivity. In general, cerium dioxide nanoparticles attach themselves to the outer surface of the animals and are taken up via the gut. However, during development water fleas molt several times leading to a loss of adherent nanoparticles. Chronic exposure with ceria nanoparticles over 21 days caused mortality and thus a reduced survival rate of the water fleas. However, this was caused indirectly by the particles, as their presence in the gut restricted the food intake [2,3,7-10]. Brine shrimp were unaffected by cerium dioxide nanoparticles at environmentally relevant concentrations [28].
Mussels display no toxic effects when exposed to environmentally relevant concentrations (PEC value) of cerium dioxide nanoparticles present in the water and excrete them without showing any impact on their viability. However, some studies found indications for changes in digestive glands, the blood composition as well as immune cells in mussels and sea urchin, respectively [29-31].
Ceria nanoparticles caused oxidative damage leading to a reduced life expectancy in roundworms (nematodes). The growth during development was also inhibited. Upon coating with positively charged materials, toxicity of cerium dioxide nanoparticles increases due to enhanced uptake. Earthworms show alterations in gut and skin tissue after exposure to cerium dioxide nanoparticles. Humic acids, however, as present in soils, can reduce the toxicity [32-35].
After uptake from the water, cerium dioxide nanoparticles end up in the liver of zebrafish. Ingested cerium dioxide nanoparticles induced growth inhibition, impaired development and physiological functions in zebrafish and goldfish. However, neither isolated rainbow trout liver cells nor zebrafish embryos showed any adverse effects after exposure with ceria nanoparticles. Equally, all observed toxic effect caused by cerium dioxide particles occurred size-independent [1-7].
The effects of cerium dioxide nanoparticles on various plants were assessed by parameters such as germination, root growth and fruit growth. Many plants internalised the cerium dioxide nanoparticles into roots and shoots. Despite particle incorporation, most plants showed normal germination but an increase in root growth. In some plants, exposure to cerium dioxide nanoparticles leads to a reduction in fruit number. Notably, cerium dioxide nanoparticles influence the composition of plants (e.g. of seeds). Probably, a change in the activity of various metabolic enzymes is responsible for this effect. Further, it has been shown that cerium dioxide can be transferred and bioaccumulate along the terrestrial food chain: zucchini-crickets-spiders [36-51].
According to the European Union legislation cerium dioxide has been classified as potentially chronically harmful to crustaceans, and potentially chronically toxic to algae, while they were not recognised as very toxic [52].
In conclusion, the toxicity of cerium oxide nanoparticles towards environmental test organisms is considered to be low. However, in some cases the adsorption of cerium dioxide nanoparticles on the surface of the organism was found to be high which could potentially lead to some adverse sub-lethal effects.
Literature
- Johnston, BD et al. (2010), Environ Sci Technol, 44(3): 1144-1151.
- Gaiser, BK et al. (2009), Environ Health, 8 Suppl 1(Suppl 1): S2.
- Van Hoecke, K et al. (2009), Environ Sci Technol, 43(12): 4537-4546.
- Jemec A et al (2015), Sci Total Environ, 506-507: 272-278.
- Lin S et al. (2014), ACS Nano, 8 (5): 4450-4464.
- Xia J et al. (2013), Biomed. Environ Sci, 26 (9): 742-749.
- Park, B et al. (2007), Part Fibre Toxicol, 4(1): 12.
- Auffan M et al. (2013), Water Res, 47: 3921-3930.
- Artells E et al. (2013), PLoS ONE, 8 (8): e71260.
- Thill, A et al. (2006), Environ Sci Technol, 40(19): 6151-6156.
- Limbach, LK et al. (2008), Environ Sci Technol, 42(15): 5828-5833.
- Garcia-Saucedo C et al. (2011), J Hazard Mater 192: 1572-1579.
- Zeyons, O et al. (2009), Nanotoxicology, 3(4): 284-295.
- Garcia A et al. (2012), J Hazard Mater, 199-200: 64-72.
- Antisari LV et al. (2013), Soil Biol Biochem, 60: 87-94.
- Bandyopadhyay S et al. (2012), J Hazard Mater, 241-242: 379-386.
- Shah V et al. (2012), PLoS ONE, 7 (10): e47827.
- Rodea-Palomares I et al. (2012), Aqua Toxicol, 122-123: 133-143.
- Röhder LA et al. (2014), Aqua Toxicol, 152: 121-130.
- Taylor NS et al. (2016), Nanotoxicology, 10(1): 32-41.
- Rodea-Palomares I et al. (2011) Toxicol Sci, 119 (1): 135-145.
- Manier N et al (2013), Environ Pollut, 180: 63-70.
- Booth A et al. (2015), Sci Total Environ, 505: 596-605.
- Van Hoecke K et al. (2011), Environ Pollut, 159: 970-976.
- Angel BM et al (2015), Aquat Toxicol, 168: 90–97.
- Rogers, NJ et al. (2010), Environ Chem, 7(1): 50-60.
- Conway JR et al. (2014), Environ Sci Technol, 48: 1517-1524.
- Gambardella C et al. (2014), Environ Monit Assess, 186: 4249-4259.
- Garaud M et al. (2015), Aqua Toxicol, 158: 63-74.
- Falugi C et al. (2012), Marine Environ Res, 76: 114–121.
- Zang H et al. (2011), Environ Sci Technol, 45: 3725-3730.
- Arnold MC et al. (2013), Arch Environ Contam Toxicol, 65: 224-233.
- Lahive E et al. (2014), Environ Chem, 11: 268-278.
- Collin B et al. (2013), Environ Sci Technol, 48: 1280-1289.
- Lopez-Moreno, ML et al. (2010), Environ Sci Technol, 44(19): 7315-7320.
- Zhao L et al. (2012), J Hazard Mater, 225-226: 131-138.
- Hernandez-Viezcas JA et al. (2013), ACS Nano, 7 (2): 1415-1423.
- Majumdar S et al. (2014), J Hazard Mater, 278: 279-287.
- Morales MI et al. (2013), J Agric Food Chem, 61: 6224-6230.
- Rico CM et al. (2013), J Agric Food Chem, 61: 11278-11285.
- Trujillo-Reyes J et al. (2013), J Hazard Mater, 263: 677-684.
- Rico CM et al. (2015), Appl Spectrosc, 69 (2): 287-295.
- Zhao L et al. (2015), Environ Sci Technol, 49: 2921-2928.
- Schwabe F et al. (2013), Chemosphere, 91: 512-520.
- Rico CM et al. (2014), J Agric Food Chem, 62: 9669-9675.
- Rico CM et al. (2015),Environ Sci Pollut Res, 22: 10551-10558.
- Corral-Diaz B (2014), Plant. Physiol Biochem, 84: 277-285.
- Zhang P et al. (2013), Nanotoxicology, 9 (1): 1-8.
- Rico CM et al. (2013), Environ Sci Technol, 47: 5635-5642.
- Tumburu L et al (2015), Environ Toxicol Chem, 34 (1): 70-83.
- Hawthorne J et al (2014), Environ Sci Technol 48:13102-13109.
- Juganson K et al (2015), Beilstein J Nanotechnol, 6: 1788-1804.
Ceria nanoparticles can be deposited in the lungs. Adverse effects in the organism only occur at very high concentrations.
Intravenous Administration and Distribution in the Body
There are only a few studies on the intravenous application of cerium dioxide [1-4]. Although the CeO2 nanoparticles have been injected into the blood stream the main portion is excreted via the faeces. However, a much larger part of the applied dose remains within the body compared to the other exposure scenarios. As most other xenobiotics cerium dioxide can be found in the liver and the spleen as has been shown by all cited studies [1-4]. Only a small amount could be found in the kidneys or the lung, within the brain and the heart the CeO2 was below the detection limit. One of these studies described a very interesting observation. After co-treatment with the liver toxin carbon tetrachloride (CCl4) cerium dioxide could prevent most of the oxidative damage by CCl4 because of its antioxidative capacity. In this case cerium dioxide has a real positive effect on the organism [1].
The other studies could demonstrate for very small particles (5 nm) [2, 3] as well as for the larger nanoparticles (30 nm) [4] an oxidative damage in the liver and partly in other organs as well. This effect was not severe but significant. It must be stated that in contrast to the study made by Hirst and colleagues [1], who have used an overall dose of 0.5 mg/kg body weight, all other three studies used very high concentration from 85 to 100 mg/kg body weight. Thus, it is no wonder that the liver shows negative effects after such high doses directly applied into the blood stream.
Literature
- Hirst, S.M. et. al. (2013), Environ Toxicol, 28(2): 107-118
- Hardas, S.S. et. al. (2012), Neurotoxicology, 33(5): 1147-1155
- Tseng, M.T. et al. (2012), Toxicol. Appl. Pharmacol., 260(2): 173-182
- Yokel, R.A. et. al. (2012), Toxicol Sci, 127(1): 256-268
Behaviour at the Blood-Brain Barrier
Cerium dioxide nanoparticles can not reach the brain after inhalation of these particles [1]. This result has been confirmed in two independent studies which have been carried out in the USA with two different variants of CeO2[2, 3]. Very small particles (5 nm) and larger ones (30 nm) have been used to inject rats with relatively high doses to investigate the distribution within the organism. Neither the small particles nor the larger ones could be found in the brain. As described by many other studies most of the applied particles could be found in the liver, the spleen and the kidneys. (read also body barriers – "Nanoparticles at the Blood-Brain Barrier").
Literature
- NanoCare 2009, Final Scientific Report, Dechema e.V., Frankfurt a.Main. ISBN 978-3-89746-108-6. (PDF-Document, 19 MB ).
Behaviour of Uptake in Somatic Cells
Data from an early study demonstrate the uptake of cerium dioxide nanoparticles between 20 and 50 nm in size by human lung cells. After the uptake the particles could be found agglomerated within vesicles in the cytoplasm of the cells. Neither outside the vesicles nor within other cell compartments such as the nucleus or the mitochondria particles could be seen [1].
Literature
- Limbach, LK et al. (2005), Environ Sci Technol, 39(23): 9370-9376.
The environmental behaviour of cerium dioxide nanoparticles (CeO2) is not well known. However, they are subject to various transport and transformation processes, which significantly influence the nanoparticles' behaviour in the respective environmental compartment water, soil and air.
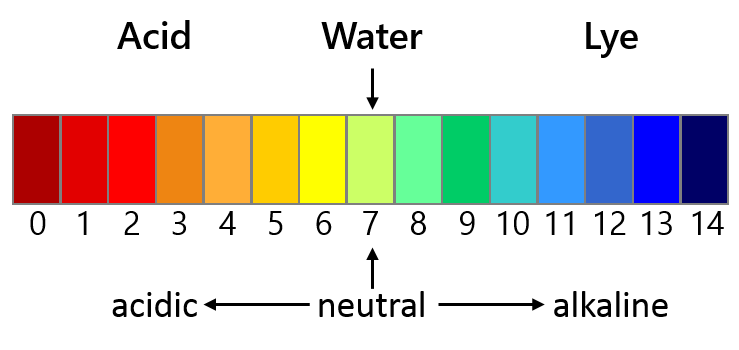
pH-scale (c) DaNa Team
Under the prevailing conditions in natural waters, these particles do not release ions. However, the solubility of cerium dioxide depends on the pH value of the respective environment. Under acidic conditions (pH values lower than 5), which occur in some natural water bodies and in acid rain, ion release increases drastically. Likewise, the mobility of ceria nanoparticles depends on pH. An acidic environment immobilises the nanoparticles and they adsorb to other materials. In contrast, under neutral or basic conditions, which prevail in most European water bodies, mobility is increased and the transport of the nanoparticles is promoted by natural organic matter [1-3].
With regard to soil, the soil composition as well as nanoparticle coating affects the mobility. The cerium dioxide nanoparticles show a strong affinity for phosphates (e.g. component of fertiliser) increasing the mobility of ceria nanoparticles [4,5].
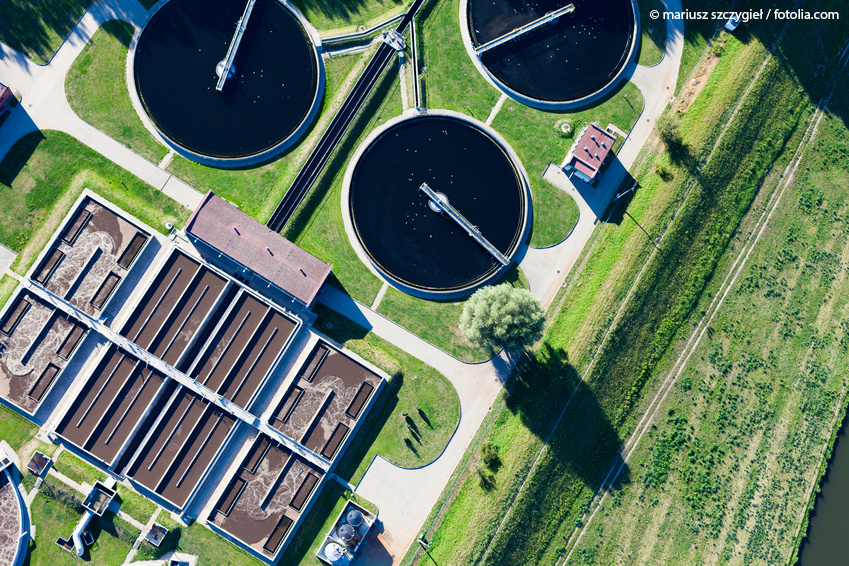
wastewater treatment plant © MariuszSzczygie / fotolia.com
In a laboratory study, a model sewage treatment plant was used to investigate the removal of metal oxide nanoparticles from wastewater. In most cases, the nanoparticles were efficiently removed from the wastewater by directly binding to the sewage sludge. Only 6 % of the original cerium dioxide nanoparticles remained in the water and ended up in the effluent in this laboratory study. In Germany, sewage sludge is either incinerated or spread on agricultural land, which is why the soil compartment is thought to be the predominant sink (see article "cerium dioxide - exposure environment").
However, nanoparticle suspensions with stabilising ingredients (e.g. humic acids) pose a greater challenge for the wastewater treatment. The nanoparticles are difficult to remove since the stabilisation hinders the binding of the nanoparticles to the bacteria in the sewage sludge. Moreover, the different composition of wastewater helps to stabilise cerium dioxide nanoparticles causing a lower sedimentation tendency compared to pure water [6,7].
Currently, there is no information available with regards to the behaviour of ceria nanoparticles upon release into the air. For e.g. diesel exhaust fumes a wash-out of the particles by rain is expected .
Overall, cerium dioxide nanoparticles do not release ions under prevailing environmental conditions. The mobility of unmodified ceria nanoparticles is low, and the majority is removed by conventional wastewater treatment processes.
Literature
- Dahle J et al. (2015), Chemosphere, 119: 1365-1371.
- Li, Z et al. (2011), Water Res, 45: 4409-4418.
- Petosa, AR et al. (2013), Water Res, 47: 5889-5900.
- Cornelis, G et al. (2011), Environ Sci Technol, 45, 2777–2782.
- Quik, JTK et al. (2010), Chemosphere, 81: 711-715.
- Limbach, LK et al. (2008), Environ Sci Technol, 42(15): 5828-5833.
- Dahle J et al. (2015), Chemosphere, 119: 1365-1371.
- Gomez-Rivera F et al. (2012), Bioresour Technol, 108: 300-304.